Methods to evaluate enzyme performance
- Like
- Digg
- Del
- Tumblr
- VKontakte
- Buffer
- Love This
- Odnoklassniki
- Meneame
- Blogger
- Amazon
- Yahoo Mail
- Gmail
- AOL
- Newsvine
- HackerNews
- Evernote
- MySpace
- Mail.ru
- Viadeo
- Line
- Comments
- Yummly
- SMS
- Viber
- Telegram
- Subscribe
- Skype
- Facebook Messenger
- Kakao
- LiveJournal
- Yammer
- Edgar
- Fintel
- Mix
- Instapaper
- Copy Link
Posted: 15 December 2010 | Sarab Sahi, Principal Research Officer, Baking & Cereal Processing Department, Campden BRI | No comments yet
Enzymes are widely used in the cereals industry to improve processing performance1 and product quality in a range of food products. Industrial baking in particular uses microbial enzymes as processing aids to improve dough properties as well as to improve product quality and shelf-life, and is an area where there is strong growth. Campden BRI is particularly interested in enzymes that have been developed to improve the baking process. Examples of enzymes investigated in research programmes have included xylanases, lipases, amylases, proteases and a number of oxidases. In this respect, Campden BRI plays an important role for its industrial member companies and the wider industry in evaluating the functional improvements from adding enzymes. There is usually only a limited amount of information available in the literature about the effects of these enzymes and it is not possible to deduce from published sources whether this is applicable across all bakery products or how they should be used most effectively.
Enzymes used in the processing of cereal products are classed as exogenous, as opposed to the endogenous enzymes that occur naturally within the cereal grain. Added or exogenous enzymes are finding more and more applications; for example, they are being used as alternatives to chemical additives2 or to improve the nutritional quality of a food. One of the issues facing the end users of an exogenous enzyme is how much to use and how effective it is likely to be when used in a specific product system. It is not an easy task to determine the concentration of an added enzyme. It is likely to be present in very small amounts and factors such as purity and potential inhibitors of activity present in wheat flour can hamper attempts to quantify activity accurately.
The declared activity of enzymes from suppliers is a good starting point, but does not tell the whole story when recipe formulations are being considered. This is because the suppliers of enzymes specify performance in terms of activity under idealised conditions. These can be far removed from a real food system in terms of pH, temperature, substrate concentration and the presence of other ingredients such as fat and sugar that can also impact on activity. Therefore, any number of ingredients and/or environmental factors can have a limiting effect on enzyme performance, which may not produce the expected result in processing operations or achieve expected benefits to quality and shelf-life of a product. It is therefore essential that when exogenous enzymes are being used, they are evaluated under conditions that reflect as close as possible relevant recipe and processing conditions.
At Campden BRI, a comprehensive approach has been adopted to evaluate novel enzyme performance. This broadly consists of characterising the performance and side activities in a lean formula followed by full recipe formulation and appropriate processing and baking trials in pilot bakeries. This approach has been applied to recent studies in commercial xylanases and lipases.
Xylanases
Xylanase enzymes, also known as pentosanases, are a group of enzymes that can break down arabinoxylans and are used in breadmaking to improve volume and crumb quality. A number of xylanases were investigated and their performance evaluated in flours of different breadmaking properties. Initial trials with each enzyme were carried out in dough systems based on flour, water, salt and fat and the doughs were mixed to a standard work input of 11Wh/kg. The physical properties of the dough were tested using a strain-controlled rheometer fitted with parallel plate geometry (diameter 25 millimetres and a gap of one millimetre). Rheological testing was performed on the doughs straight after mixing and after storing at 30°C at 15 minute intervals, up to 75 minutes in total. The elastic modulus G’ (which is a measure of the stiffness of the dough) at one hertz was used to follow the activity of the enzyme with time, and compared with that obtained at time t = 0 so that the difference ΔG’ could be plotted against time. The value of ΔG’ was positive or negative depending on whether the dough was becoming stiffer or softer with time.
This is demonstrated in Figure 1, which shows the change in the elastic modulus for the control dough and one with added xylanase. For 20 minutes, the control dough became stiffer after mixing as a result of damaged starch absorbing water slowly, a phenomenon known as ‘cold swelling’. The modulus then fell and crossed the zero line (same modulus as at zero time) after 38 minutes, after which there was a gradual softening of the dough as a result of the hydrolytic activity of various enzymes naturally present in the flour. The elastic modulus continued to fall before levelling off, presumably due to the exhaustion of the substrate of the enzymes concerned. With the addition of a commercial xylanase, the elastic modulus falls below the value of the control dough after 15 minutes and then continues to fall with time as a result of the hydrolysis of the non-starch polysaccharides (arabinoxylans) naturally present in the flour.
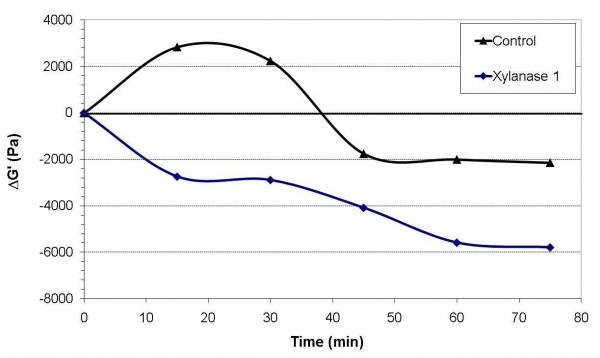
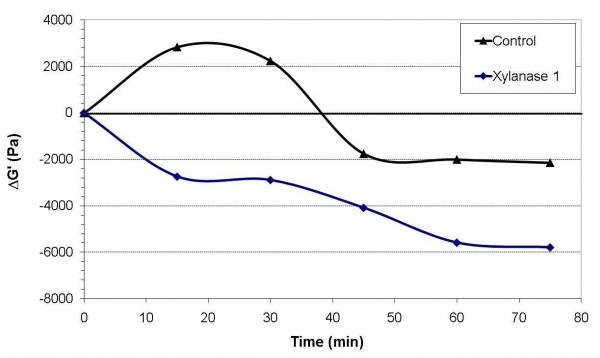
Figure 1 Example of rheological changes with xylanase addition in a basic dough system
The rate of change in the stiffness of the dough with time can be used as a measure of the activity of an enzyme preparation in a particular system and can be used as the basis of a rapid method to screen the activity of enzymes such as xylanases or other hydrolytic enzymes that cause changes in the rheological properties of dough systems. This type of measurement provides a rapid assessment of the performance of the enzymes, which can then be further backed up by evaluation in full recipe dough system with pilot-scale processing similar to commercial practice.
The results of measurements on full recipe doughs (Figure 2) show the response of the dough elastic modulus to increasing the concentration of a xylanase after mixing and after a period of proof. The results clearly show the level of addition of the enzyme required to cause significant changes in the stiffness of the dough and the effect of further additions. A key requirement when using xylanases in dough systems is to obtain controlled action in the dough to provide optimum rheological changes in the dough and obtain the desired volume and crumb quality. High level of addition of a xylanase can make the dough too soft and sticky and this is not desirable in a plant bakery as problems occur during handling of the dough that can lead to damage in the moulding operations. This is most noticeable in the final moulding, leading to loss in product quality. This type of rheological measurement allows informed decisions to be made about the level of use of specific enzymes in particular recipe systems. The measurements made on the dough after proof are also very meaningful as the dough has increased in volume as a result of yeast fermentation and has become soft and hence susceptible to damage during mechanical handling when the dough is transferred from the proving cabinet and into the oven. The data in Figure 2 indicates that high levels of this particular xylanase may cause the dough to become too soft and even collapse as a result of mechanical handling during transfer. This may also happen if there is a delay during processing and the floor time is increased, allowing more time for the enzyme to act in the dough.
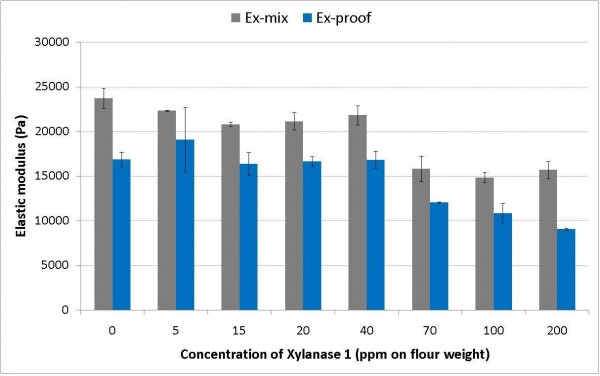
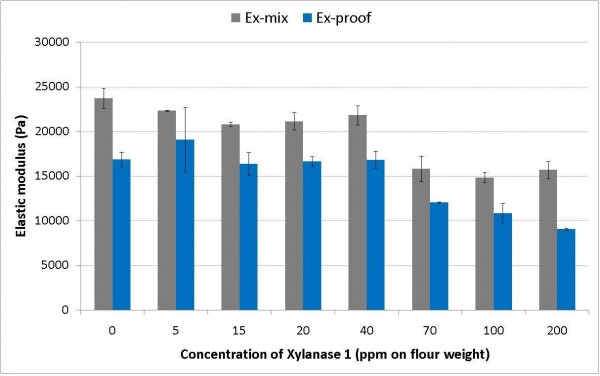
Figure 2 Effect of xylanase 1 addition on the elastic modulus of a full recipe CBP dough
Ultimately, the best way to elucidate the impact of an enzyme on product quality is to actually perform baking trials. This was done to determine the effect of xylanases on bread volume and crumb quality. The Chorleywood Bread Process method was used to make 800 gram loaves. The benefits of carrying out a dose versus volume response can be clearly seen in Figure 3.
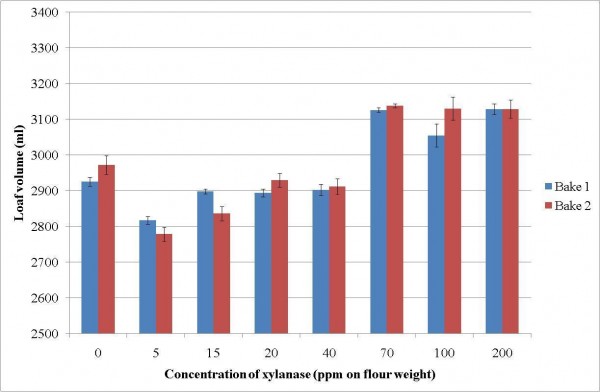
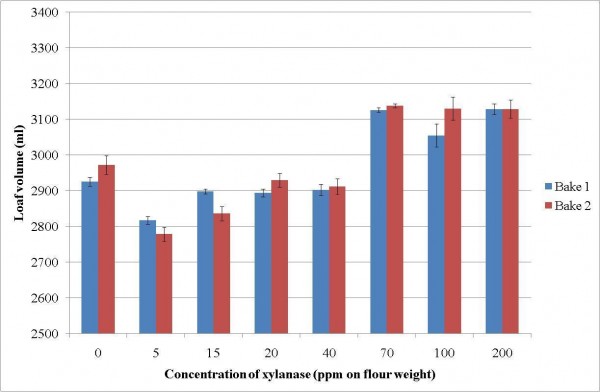
Figure 3 Effect of xylanase 1 addition on the volume of the bread
Results show that there was an optimum level of use of the enzyme to give an improvement in volume (Figure 4) and this coincided with the rheological change in the dough properties shown in Figure 3 on page 36. Clearly, additions greater than 70 ppm do not provide any further benefits to the volume of the bread, but may result in the doughs becoming soft and sticky.
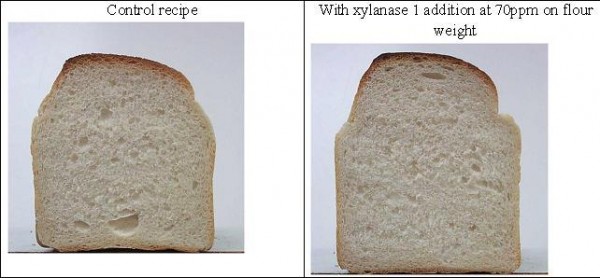
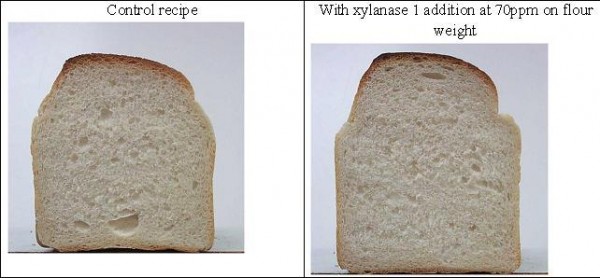
Figure 4 Improvement in loaf volume and crumb structure with xylanase addition
Lipases
Whereas xylanses have a measurable effect on dough rheology, the detection of action of other enzymes is not so straightforward. For example, for enzymes such as lipases, the action of which generates materials similar to widely used emulsifiers in baking, the measurement of changes in dough systems can be challenging as it is not always possible to obtain clear evidence of their functionality using rheological methods. Consequently, the action of lipases added to modify lipid substrates naturally present in flour as well as ingredients such as bakery fat and egg is not straightforward to characterise. An interesting way to get around this problem is to make use of the fact that the surface activity of the materials produced by lipase action is generally greater. That is, the new material produced after cleaving a fatty acid chain from the native lipid molecule is able to reduce the interfacial tension more than the original molecule before the enzyme was added. This approach has been used at Campden BRI to screen lipase activity against different substrates such as whole egg and heat-treated and high protein flours.
Three lipases were added to liquid whole egg and the interfacial tension was measured on a Krüss drop shape analysis system (DSA 100). This technique analyses the shape of the pendant drop and calculates the interfacial tension between the two phases (egg solution and air) knowing their densities. The measurements were performed on drops as soon as they were formed and the surface tension was calculated according to the Young-Laplace method.
The results of adding lipases to whole egg (Figure 5) shows that only one of the lipases (lipase 3) reduced the surface tension of the egg-air interface and continued to do so during the measurement. The change in the surface tension was indicative of the fact that surface active material was generated as a result of lipase action, for example, the lecithin in the egg yolk being converted to lysolecithin. Lipase 1 and 2 addition had no effect on the surface tension, suggesting either low activity of these enzymes or lack of suitable substrate.
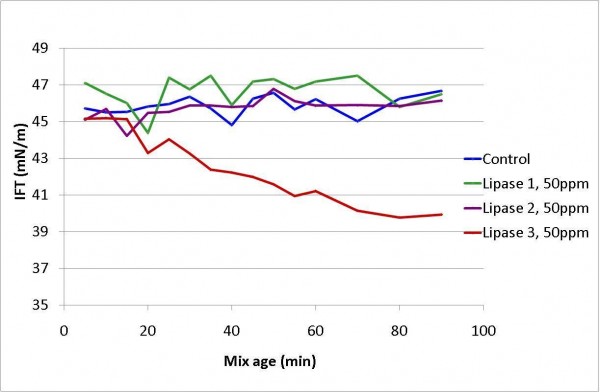
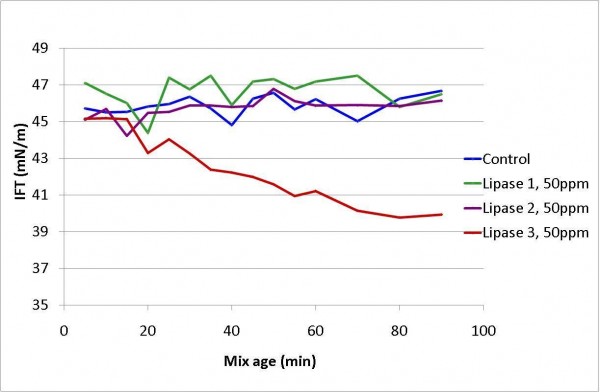
Figure 5 Change in interfacial tension at the air-liquid egg interface with lipase addition
The addition of lipases to the flour-water system (Figure 6) shows that all three enzymes were active, with the surface tension decreasing with time and the biggest reduction achieved by lipase 3. In wheat flour, the lipids make up one to two per cent of the flour weight and consist of both non-polar (triglycerides) and polar (glycolipids and phospholipids) lipids in roughly equal amounts. The results, therefore, suggest that there was suitable substrate in the flour and that the activity of the enzymes can be assessed by following the change in the surface tension. The results also suggest that if a novel enzyme is being developed for a specific lipid substrate, this type of test will confirm the presence or absence of the appropriate activity.
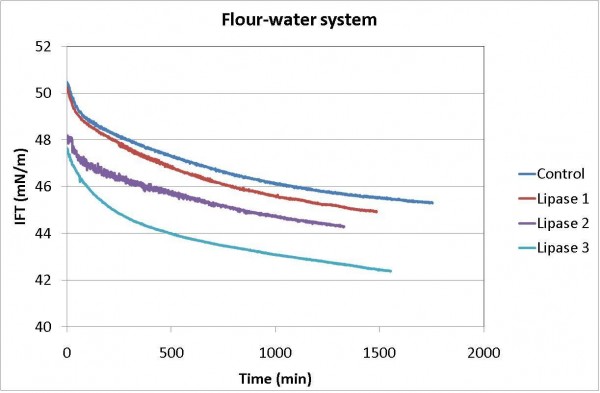
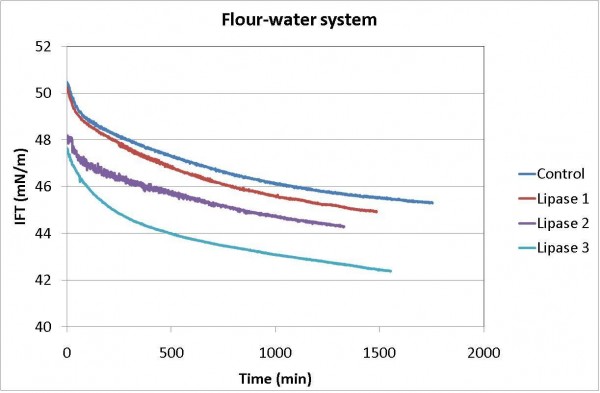
Figure 6 Change in surface tension of flour-water system with lipase addition
Conclusions
The activity of enzymes such as xylanases that hydrolyse arabinoxylan chains in a dough system can be evaluated by measuring the change in the physical properties using rheological measurements. Such measurements can be utilised to optimise the time / temperature impact of enzyme activity in specific flour based systems. Enzymes showing the required activity against key ingredients can then be further evaluated using full recipe formulations and processing conditions to fully optimise dosage and functionality. For enzymes such as lipases, it has been demonstrated that surface tension measurements can be used to determine exogenous lipase activity in both whole egg and flour-water systems. This offers enzyme manufacturers, ingredient suppliers and end users a rapid route to evaluate the likely performance of lipases in specific systems. The technique could be used most effectively at the enzyme development stage to rapidly screen enzymes with the most promising activity and hence speed up the selection process.
References
1. Poutanen, K. (1997). Enzymes: An important tool in the improvement of the quality of cereal foods. Trends in Food Science & Technology, 8, 300-306
2. Guy, R.C.E. & Sahi, S.S. (2006). Application of a lipase in cake manufacture. Journal of the Science of Food and Agriculture, 86, 1679-1687
About the Author
Sarab Sahi is Principal Research Officer in the Baking & Cereal Processing Department at Campden BRI. Sarab is a graduate in Biochemistry and has a PhD in physical chemistry from Reading University. After a stint as a postdoctoral fellow investigating protein/lipid interactions, he has worked in the cereal sector for over 20 years. He has specialist knowledge in physical measurements and application of enzymes and emulsifiers in bakery foods. Sarab’s current research interests include natural materials with redox properties for bakery systems as well as application of interfacial and bulk rheological measurements to understand key ingredient functions in bakery systems.