Using mechanical testing to replicate the sensory profile of meats
- Like
- Digg
- Del
- Tumblr
- VKontakte
- Buffer
- Love This
- Odnoklassniki
- Meneame
- Blogger
- Amazon
- Yahoo Mail
- Gmail
- AOL
- Newsvine
- HackerNews
- Evernote
- MySpace
- Mail.ru
- Viadeo
- Line
- Comments
- Yummly
- SMS
- Viber
- Telegram
- Subscribe
- Skype
- Facebook Messenger
- Kakao
- LiveJournal
- Yammer
- Edgar
- Fintel
- Mix
- Instapaper
- Copy Link
Posted: 6 August 2021 | Mark Auty, Robert Gurney | No comments yet
Mark Auty and Robert Gurney look at the use of mechanical testing to characterise the properties of meat alternatives and their impact on sensory profile.
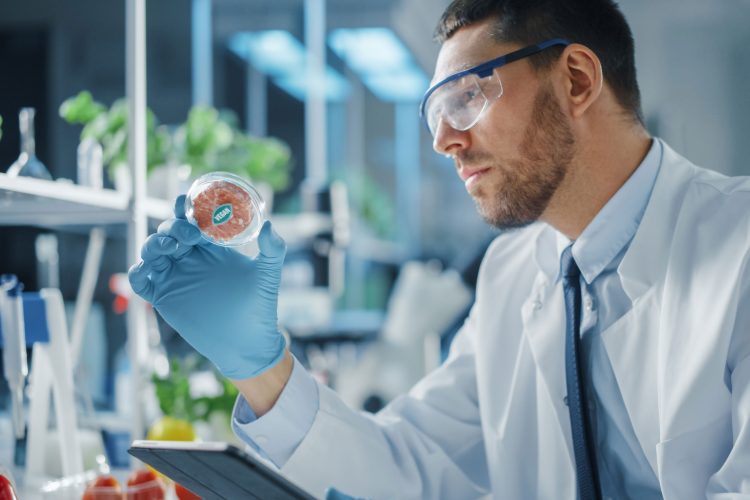
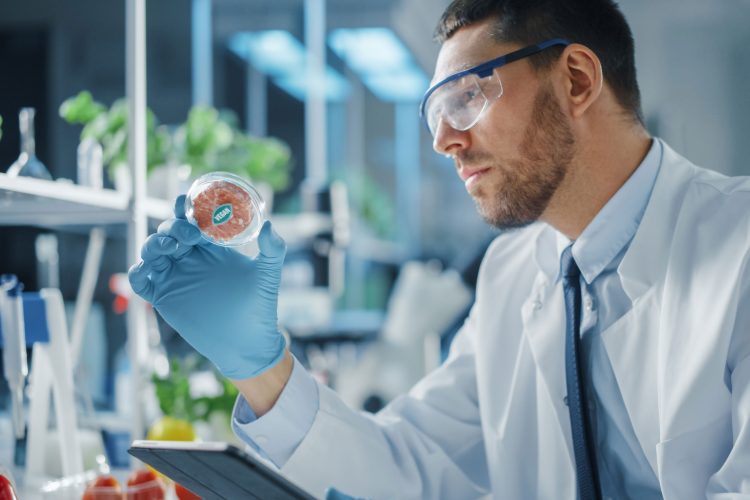
As more and more consumers consider the health, environmental and ethical impact of their food choices, meat consumption is increasingly coming under the microscope. While around 14 percent of adults (7.2 million) in the UK are following a meat-free diet,* many more flexitarians are emerging – those who intentionally only consume animal-based foods in moderation.
The number of Brits who have eaten meat-free foods increased from 50 percent in 2017 to 65 percent in 2019 (Mintel).1 However, with growing numbers of consumers becoming vegetarian or vegan and more meat eaters regularly choosing plant-based alternatives, these products must provide a fulfilling and tasty eating experience to capture the attention of a more diverse and discerning consumer-base.
Meat alternatives have come a long way since meat was rationed during and after the Second World War; in 2019, the UK meat alternative market was valued at $489.2 million and is expected to hit $726.8 million in 2025, increasing at an estimated annual growth rate of 6.8 percent between 2020 – 2025, according to The Vegan Society.
In this fast-paced and lucrative market, food developers need to create exciting meat-free products that offer both nutrition and great taste – but it is notoriously difficult to replicate the sensory profile of meats. We look at how researchers use mechanical testing to characterise foods and how these properties correlate with the eating experience.
Analysing the mechanical properties of meat alternatives
Tasked by a challenger meat alternative brand to compare properties of chicken with new chicken alternatives, scientists at Reading Scientific Services (RSSL) set about examining their structures while performing tensile testing. They were able to relate these properties to sensory profiles, including taste, smell and, importantly, texture, to discover which physical properties most impacted the eating experience.
Mechanical testing is a popular characterisation technique that is used across almost all research fields, from understanding the behaviour of graphene nano-threads to steel-reinforced concrete beams, or from the elasticity of rubber tyres to the flexibility of muscle fibres. Researchers have a wide range of tensile and compressive tests that can be used to identify intrinsic properties of these materials, including food.
Tests to characterise the chicken alternatives were chosen in order to best relate to the sensory experience, in particular the food texture. Tests were performed using a mechanical testing device (Linkam MFS – Modular Force Stage) and some of the parameters that were calculated – all of which relate to sensory experience – included:
- Extensivity and failure mode – how far the sample stretches before failing, and the failure mode – ie, clean break or fibrillar staggered breakage
- Hardness – how much force is required to break the material
- Young’s Modulus – a measure of the stiffness and elasticity of a material based on its initial resistance to being stretched
- Cohesiveness and resilience – how well the product withstands a second deformation under compression (chewing) relative to its resistance under the first deformation, and how well a sample returns to its original shape after compression
- Springiness – how well a product physically springs back after it has been deformed during the first compression, ie, after one ‘chew’.
RSSL worked with Linkam Scientific Instruments to evaluate the best set-up for these mechanical tests on food. In addition to the standard mechanical testing stage, customised equipment was identified for the compression tests. As well as jointly designing the tensile test parameters for imaging the samples, they then underwent assessment using microscopy techniques within the RSSL laboratory.
Sample preparation
Chicken breast and three plant-based chicken products were used for these experiments. All of the foods were available at UK supermarkets in the chilled aisle, and all of the plant-based products claimed to be similar to chicken. The chicken breast and the three plant-based chicken alternatives (two soy protein based and one soy and pea protein based, henceforth referred to as Soy-Protein-S1, Soy-Protein-S2 and Pea-Protein-S3) were cooked according to the manufacturer’s instructions. Informal sensory tests were performed before mechanical testing commenced to understand the mouthfeel of each and examine the macro structural properties. The researchers tasted an equal size piece of each sample and commented on the texture and mouthfeel of the samples, noting their findings which are summarised in Table 1 below.
For mechanical testing, similar-sized samples were cut from the cooked foods, with a size of approximately 20x5x5mm. The samples were prepared on a supporting frame, and tensile grips were used to hold the sample assembly. The MFS was used for tensile and compressive testing at room temperature and pressure. The stage was placed under a Zeiss v16 optical zoom microscope and images were captured as the experiment took place.
Extension experiments were performed at a speed of 200µm/s. The movement direction of the grips was parallel to the fibre alignment of the chicken (and other samples where fibres were visible). The extension tests continued until the samples visibly failed and the force dropped to zero. At least three replicates of each test were carried out.
Two-cycle compression experiments were performed using similar sized samples which were loaded between the compression grips. A speed of 200µm/s was used and the plates compressed the sample by 5mm. The direction of the compression plates was perpendicular to the fibre alignment of the chicken (and other samples where fibres were visible).
Force-extension and force-time graphs were created with data from the tensile and compressive experiments respectively. From the tensile data, researchers calculated the Young’s modulus EY using the formula EY= σ/ε, where stress σ= force/sample area and strain ε=extension/initial sample length. The mechanical results are summarised alongside the sensory results in Table 1.
Results
The images captured from the extension testing showed that under tensile testing, all samples failed in a similar way with fibres breaking at the point of failure. It was interesting to note that the only the Soy-Protein-S2 had similar fine fibres to chicken (Figure 1), while the other plant-based samples did not have similar fine fibres.
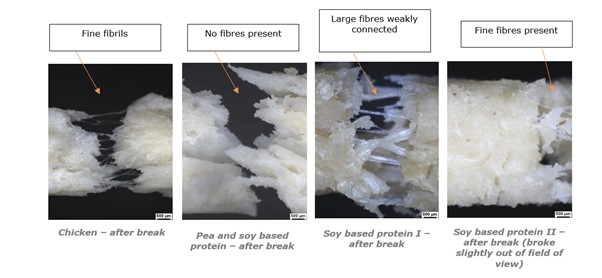
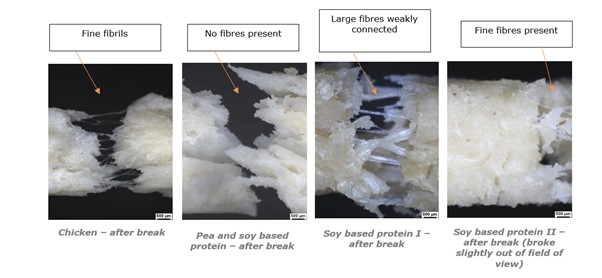
Figure 1: Images of the chicken and plant-based alternative samples captured during tensile testing just after the point of sample failure, visually showing how the samples change at their breaking point. Images captured using a Linkam MFS with a Zeiss v16 optical zoom microscope
Representative force extension curves, chosen from similar repeat experiments, are shown in Figure 2. It is noteworthy that the Soy-Protein-S2 had a similar breaking profile to chicken, with an initial yield shoulder and drop in force required to extend the sample as it began to break, followed by several smaller drops and shoulders as the fine fibres continued to break. This is in contrast to the Pea-Protein-S3 and Soy-Protein-S1, with the former failing immediately without any remaining interconnected fibres, and the latter having a less pronounced failure with no significant yield points, indicating it has more weakly connected fibres which don’t break in the same way as the fine fibres of chicken.
Figure 2: Results from tensile extension experiments showing (a) Forve vs. Extension and (b) Stress vs. Strain for each sample
The chicken showed the highest Young’s Modulus EY (Figure 3a), demonstrating that it was the stiffest of the samples, likely a result of its tight fibrous structure. Soy-Protein-S2 and the pea protein followed with moderate values of EY, while the Soy-Protein-S1 has a lower value, indicating it is not as stiff as the others. Those with similar values of EY should show a similar firmness and elasticity to chicken samples.
The Pea-Protein-S3 product (which did not have a fibrous structure), required the most force to break (Figure 3b). This was likely due to strong adhesion between the material comprising its structure, but lack of interconnecting fibres to increase the modulus, resulting in a tougher texture. The Soy-Protein-S2 and Pea-Protein-S3 samples had hardness values above and below that of chicken respectively.
Figure 3: Comparison of (a) the Young’s Modulus and (b) Hardness for the chicken and plant-based alternative samples . Results calculated from average values from the tensile experiments
The compression experiments for all samples produced relatively similar force-time profiles as seen in Figure 4. After two compression cycles, the chicken sample required the least force to compress a distance of 5mm, indicating that it had the lowest cohesiveness, and Pea-Protein-S3 required the highest force and thus had the highest cohesiveness. This indicated that the chicken structure had likely broken down most over the compression cycles.
Figure 4: Compression Tests: (a) Resilience: Force vs. Indentation distance for each of the four materials, (b) two consecutive compression tests to demonstrate cohesiveness after a second compression
Soy-Protein-S1 had the highest value for resilience, demonstrating that it sprung back quickly after deformation and was likely to have a more rubbery/chewy texture. Pea-Protein-S3 and Soy-Protein-S2 took the most force to compress. This correlates with similar results for hardness found in the extension testing.
While none of the chicken alternative products consistently had similar textural properties to the chicken, the Soy-Protein-S2 product showed the most similar profile. This was considered likely due to the presence of fine fibres in its structure, similar to chicken fibrils.
The results from the sensory tests are compiled in Table 1. Sensory testing showed that the chicken sample had a chewy, moist mouthfeel, which corresponds to its fine fibrous structure that yielded the highest Young’s Modulus in mechanical testing.
In tensile testing, the Pea-Protein-S3 failed immediately without any remaining interconnected fibres and displayed the highest cohesiveness and hardness, resulting in its firm texture in the sensory testing.
Soy-Protein-S1 had more weakly connected fibres which don’t break in the same way as the fine fibres of chicken, plus the lowest Young’s Modulus, resulting in its chewy texture.
Soy-Protein-S2 provided a texture that was most similar to chicken, due to it having similar fine fibres and a similar breaking profile. It was found to be harder and required more force to compress than chicken, which may correspond to the lower moistness perception.
Specimen type and image |
Physical appearance |
Sensory description of texture |
Mechanical properties |
Chicken
|
Skeletal muscle composed of tightly packed muscle fibres/cells which are aligned longitudinally. Each muscle fibre is composed of smaller tightly packed muscle fibrils also aligned longitudinally. This structure allows the muscle fibres to extend giving contraction and relaxation also giving a unique texture for food products. |
Chewy, moist mouthfeel typical of chicken. |
A strong and stiff material. |
|
Layers of homogeneous material containing no fibres. |
Firm, not very moist. |
A strong material but with little structure. |
|
Mix of homogeneous material and large fibres. |
Chewy texture, moderately moist. |
A weak but ductile material (due to the presence of large fibres but loosely connected). |
|
Mix of homogeneous material and small thin fibres. |
Chewy texture, moderately moist. |
A moderately strong and stiff material. |
Table 1: Summary of the sensory description of each sample’s appearance, texture and overview of the mechanical properties found from testing.
Conclusion of study
The team found that there was a strong correlation between the human sensory feedback from handling and consuming the product samples, and the data output from the tensile and compression tests. Understanding the physical and microstructural properties of food is vital in the product development process. This kind of experiment and data could help product formulators to develop meat-alternatives based on different ingredients, that mimic the eating experience of real chicken and potentially other meat and meat alternatives.
Equipment
These experiments were conducted using a MFS – a compact system with a temperature, gas and optional humidity-controlled sample environment. It was mounted on a microscope which was used to directly image changes in the microstructure of the samples while they were undergoing tensile (in-situ) testing. Correlating this optical information with the physical characteristics that were measured by the stage enabled the team at RSSL to better understand the behaviour of the samples when subjected to force
References
- Source: www.finder.com/uk/uk-diet-trends, Analysis conducted by finder.com/uk
* Including meat substitutes, or dishes made using meat substitutes, including ready meals, sausages, burgers, deli ingredients (eg, vegetarian mince), pastry products and snacks.
About the authors
Dr Robert Gurney is Marketing and Applications Specialist at Linkam. He has expertise in a range of scientific techniques for temperature, environmental and mechanical sample characterisation. Robert obtained his PhD in Soft Materials from the University of Surrey and has worked at ESPCI Paris and Wuhan University of Technology on projects, including thin film analysis, chemical synthesis, and solar cell characterisation.
Dr Mark Auty is Food Microstructure Leader at Reading Scientific Services Ltd. With a BSc in Microbiology and PhD in Dairy Chemistry (UCC), Mark has a strong research background, with over 100 scientific publications, mainly in dairy science. Research interests include relating the microstructure of food to its physico-chemical and sensory properties across all food sectors, advanced imaging systems and digitisation. Currently, he holds Fellowships with the Royal Microscopical Society and the Institute for Food Science and Technology.