Biobased lubricants – ensuring oxidative stability
- Like
- Digg
- Del
- Tumblr
- VKontakte
- Buffer
- Love This
- Odnoklassniki
- Meneame
- Blogger
- Amazon
- Yahoo Mail
- Gmail
- AOL
- Newsvine
- HackerNews
- Evernote
- MySpace
- Mail.ru
- Viadeo
- Line
- Comments
- Yummly
- SMS
- Viber
- Telegram
- Subscribe
- Skype
- Facebook Messenger
- Kakao
- LiveJournal
- Yammer
- Edgar
- Fintel
- Mix
- Instapaper
- Copy Link
Posted: 3 November 2021 | Alejandro Marangoni | No comments yet
The momentum for green chemistry and more sustainable products is gaining. Consumers are demanding more biobased, less toxic, and more ‘natural’ products, showing a definite move away from petroleum-based products, towards renewables. Here, Alejandro Marangoni explains that oxidative stability must be ensured to achieve an effective transfer.
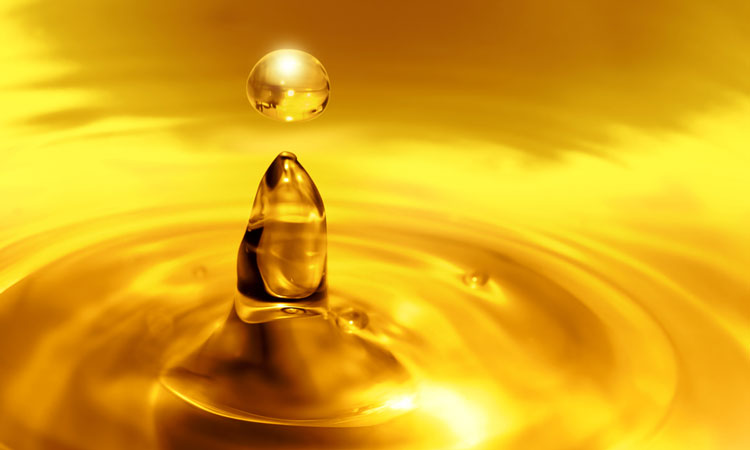
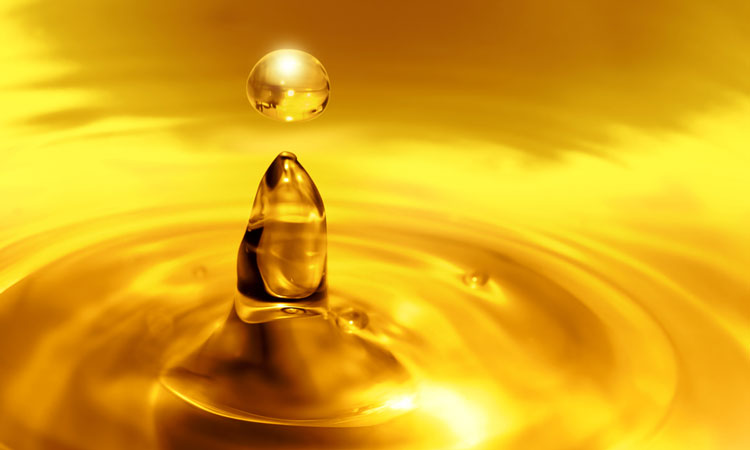
There are often no green alternatives to high performance petroleum-based products, either due to lack of performance or lack of availability and high cost. At WPL, in association with the University of Guelph, we have been developing technology behind biobased suspension oil, grease, dry and wet chain lubes, cleaners, seal conditioners, among others, since 2014. There are many performance aspects behind these materials but the main difference between any petroleum-based lubricants and biobased products is the base oil. Petroleum-based is derived by fractionating petroleum, rather than synthesis from petroleum stocks, such as synthetic oils, eg, polyalphaolefins.
The problem with mineral oils
The base oil in petroleum-derived lubricants is some version of mineral oil. Refined mineral oils are long-chain, branched, cyclic or linear, saturated aliphatic hydrocarbons. Saturated hydrocarbons contain only carbon and hydrogen in their structure and do not contain unsaturated carbon-carbon bonds. Unsaturated hydrocarbons contain one or more double bonds in their structure. The other issue is that mineral oils are complex mixtures of compounds and include aromatic molecules, which are known to be toxic, carcinogenic and mutagenic. A representative summary of some of the molecules present in mineral oils is shown in Figure 1.1 The refining process of mineral oils basically removes the aromatic hydrocabons rom the residual white oil (also referred to as paraffin oil, liquid paraffin, paraffinum liquidum, and liquid petroleum). Purity levels can reach those of synthetic oils. Fractionated mineral oil generally falls into three categories or grades, from low to high viscosity,1 making them very useful in lubricant applications. The usual size of hydrocarbons used in lubricant applications ranges from 20 to 40 carbons.
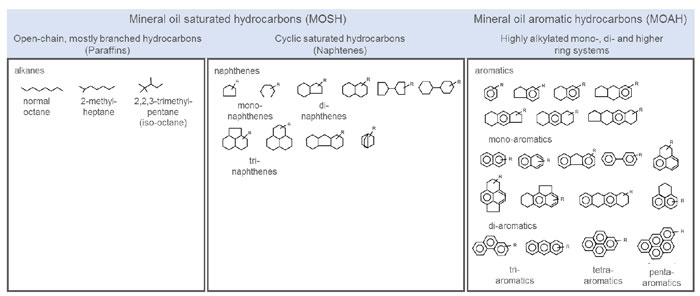
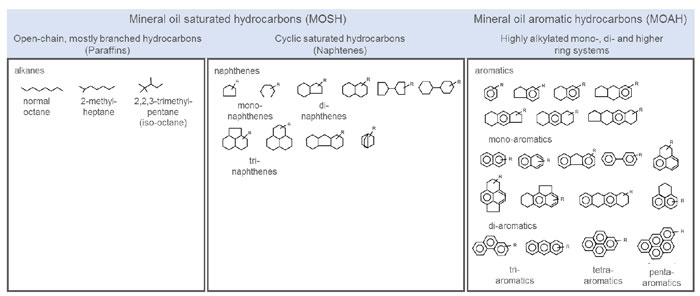
Figure 1: A representative summary of some of the molecules present in mineral oils
As summarised in the Scientific Opinion on Mineral Oil Hydrocarbons in Food EFSA Panel on Contaminants in the Food Chain (CONTAM), European Food Safety Authority (EFSA), Parma, Italy, 28 August 2013:
“Consumers are exposed to a range of mineral oil hydrocarbons (MOH) via food. Mineral oil saturated hydrocarbons (MOSH) consist of linear and branched alkanes, and alkyl-substituted cyclo-alkanes, whilst mineral oil aromatic hydrocarbons (MOAH) include mainly alkyl-substituted polyaromatic hydrocarbons. Products, commonly specified according to their physico-chemical properties, may differ in chemical composition depending on the oil source. Technical grade MOH contain 15-35 percent MOAH, which is minimised in food grade MOSH (white oils). Major sources of MOH in food are food packaging and additives, processing aids, and lubricants. Estimated MOSH exposure ranged from 0.03 to 0.3 mg/kg b.w. per day, with higher exposure in children. Specific production practices of bread and grains may provide additional MOSH exposure. Except for white oils, exposure to MOAH is about 20 percent of that of MOSH. Absorption of alkanes with carbon number above C35 is negligible. Branched and cyclic alkanes are less efficiently oxidised than n-alkanes. MOSH from C16 to C35 may accumulate and cause microgranulomas in several tissues including lymph nodes, spleen and liver. Hepatic microgranulomas associated with inflammation in Fischer 344 rats were considered the critical effect. The no-observed-adverse-effect level for induction of liver microgranulomas by the most potent MOSH, 19 mg/kg b.w. per day, was used as a Reference Point for calculating margins of exposure (MOEs) for background MOSH exposure. MOEs ranged from 59 to 680. Hence, background exposure to MOSH via food in Europe was considered of potential concern. Foodborne MOAH with three or more, non- or simple-alkylated, aromatic rings may be mutagenic and carcinogenic, and therefore of potential concern. Revision of the existing acceptable daily intake for some food-grade MOSH is warranted on the basis of new toxicological information.”
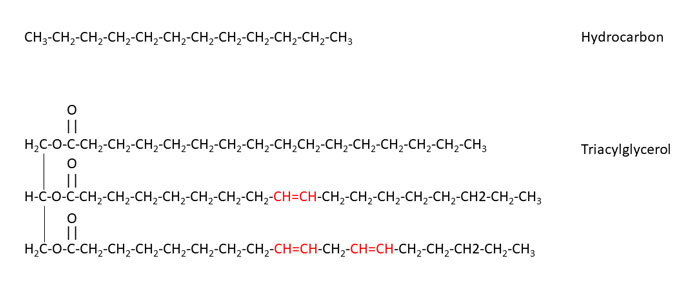
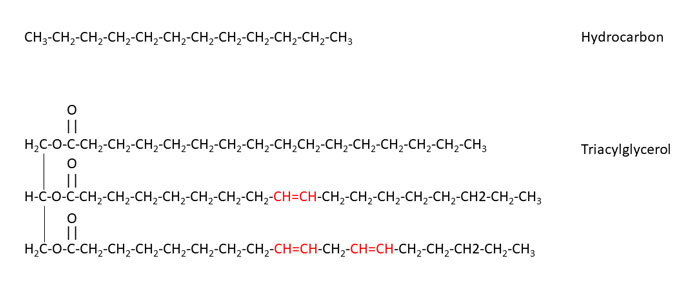
Figure 2: The chemical structures of a typical hydrocarbon, dodecane, and a typical triacylglycerol (TAG), 1-palmitoyl, 2-oleyl, 3-linoleyl glycerol
The most basic transformation of a lubricant oil, used in all lubricant, hydraulic fluid, suspension oil and grease applications, is to replace the mineral oil with a vegetable oil. Figure 2 shows the chemical structures of a typical hydrocarbon, dodecane, and a typical triacylglycerol (TAG), 1-palmitoyl, 2-oleyl, 3-linoleyl glycerol. It may appear that these molecules are very different, and that is true. While both feel similar to the touch, there are fundamental differences – the first being that TAGs are esters of glycerol and three fatty acids, while mineral oils are basically saturated hydrocarbons of differing molecular weights.
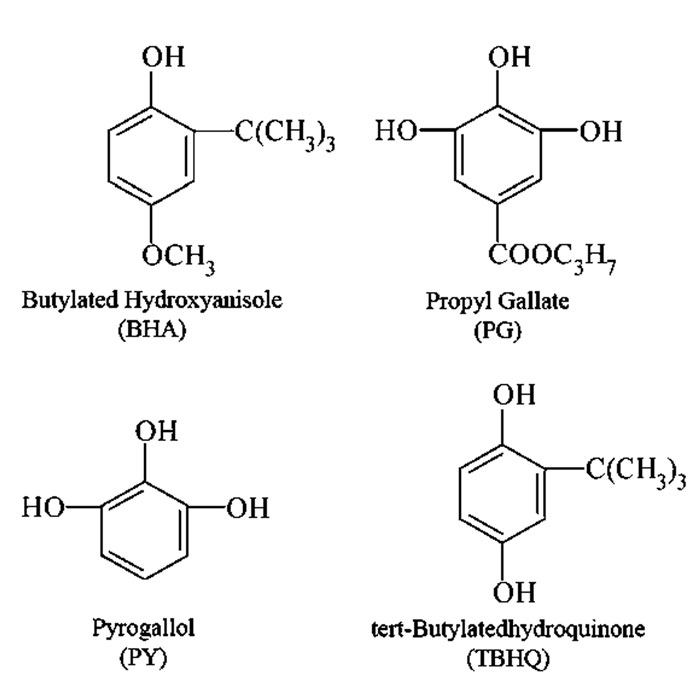
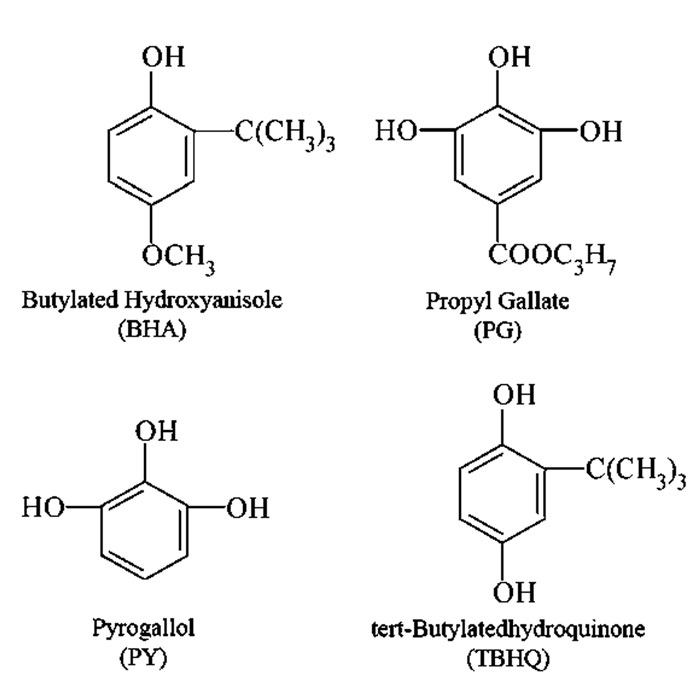
Figure 3
Saturated hydrocarbons are very resistant to degradation since there aren’t any double bonds to oxidise. However, vegetable oil TAGs have two issues. The first is the propensity of the ester bond to hydrolyse in the presence of some water and heat, enhanced by the presence of acid, causing TAGs to undergo hydrolytic breakdown into partial glycerides (mono and diglycerides, glycerol) and free fatty acids. This leads to a viscosity breakdown, increased acidity and loss of lubricating properties. Moreover, all these lower molecular weight molecules have lower boiling points and will volatalise more readily.
The oxidation issue
The second reaction, which is particularly problematic with vegetable oils, is oxidation. As you may know, the fatty acids which form part of the TAG molecule can have several unsaturations, or double bonds. Carbon-hydrogen bonds on the carbon next to the carbon-carbon double bond are very labile to homolytic cleavage, where each electron participating in a covalent bond remains with its partners upon breakage, thus forming a pair of free radicals. Oxidation is a free radical-mediated chain reaction, and once this first free radical is formed, the oxidation reaction will proceed very rapidly. One useful concept for understanding the propensity for vegetable oils to oxidise and thus degrade is that of the bond dissociation energy (BDE) of carbon-hydrogen bonds in the presence and absence of double bonds and how close they are to that double bond (Table 1). The homolytic bond dissociation energy is the amount of energy needed to break apart one mole of covalently bonded gases into a pair of radicals, and estimated from the differences in the molar heats of formation of each of the radicals minus the heat of formation of the bond.
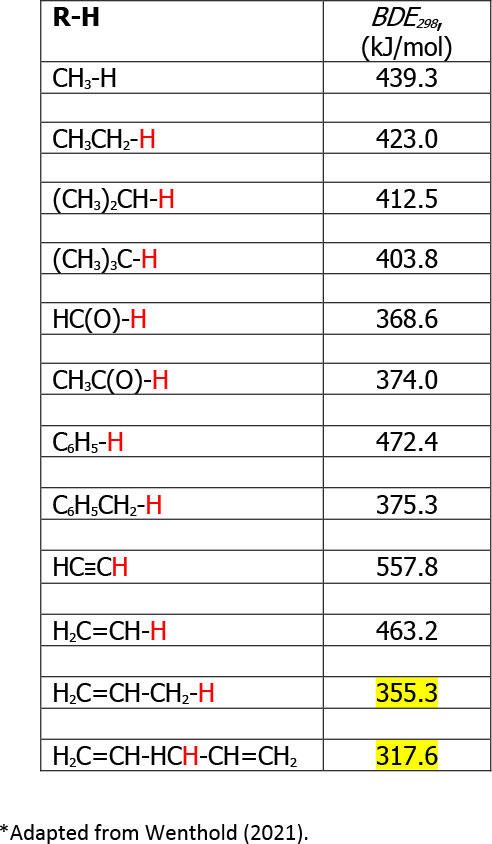
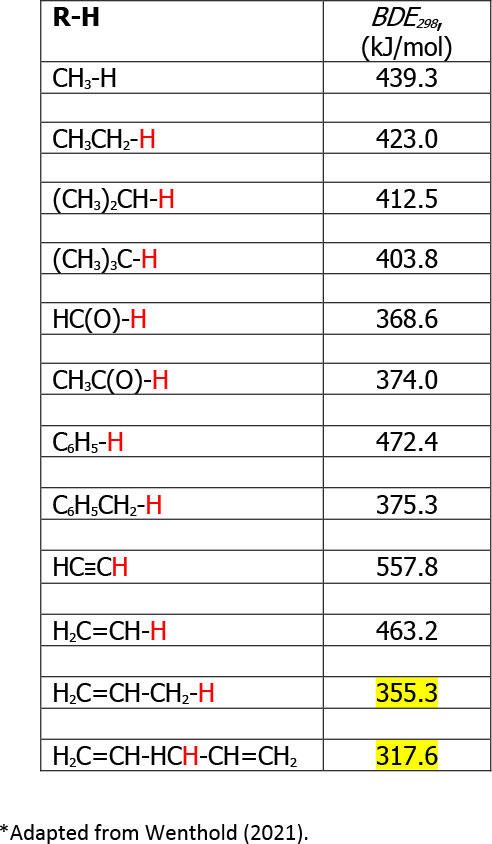
Table 1. Homolytic bond dissociation energies for carbon-hydrogen bonds at 25oC and 1atm pressure. *Adapted from Wenthold (2021).2
Table 1 illustrates how the initial homolytic bond cleavage for a C-H bond in a saturated hydrocarbon would be about 423 kJ/mol, while for a C-H bond in a polyunsaturated fatty acid of a TAG, say linoleic acid, would be 317 kJ/mol. The relative weakness of this bond is the main reason why vegetable oils oxidise far more readily than saturated hydrocarbons. Even monounsaturated fatty acids have a BDE of 355 kJ/mol, which is still much lower than that of a C-H bond in a saturated hydrocarbon.
So, when choosing a vegetable oil for a biolubricant application, one should choose an oil with a low content of fatty acids with multiple double bonds. The common polyunsaturated fatty acids in vegetable oils are linoleic and linolenic acids, with 18 carbons and two and three double bonds, respectively. As you can imagine, high linolenic acid (18:3) oils are very labile towards oxidation, with some, like linseed oil, being used as drying oils due to their propensity towards oxidation and polymerisation. Most oils contain significant amounts of linoleic acid (18:2), soybean oil being the most well known, with over 55 percent linoleic acid. This oil is also very prone to oxidative degradation. While it will not be covered here, the initial free radical initiation reaction is quickly followed by reaction with oxygen and formation of hydroperoxides (primary oxidation products). These, in turn, will homolytically cleave into alkoxy and hydroxy radicals. The alkoxy radicals will then break down and split the fatty acid chain, usually next to the original double bond, and form lower molecular weight aldehydes, acids, alcohols, among others (secondary oxidation products). As more free radicals are formed, the reaction propagates. Eventually, as all the substrate is gradually reduced, reactions between free radicals start yielding non-radical products and the reaction slowly terminates. However, one is left with a soup of lower molecular weight polar organic molecules instead of the original oil. Besides oxidation and eventual breakdown, another prominent free radical-mediated reaction takes place at the same time; polymerisation. In the absence of oxygen, the free radicals generated in the initiation stage of the auto-oxidation process will just react with other free radicals; some of them from neighbouring TAGs. This will create dimers, which can eventually create TAG oligomers. If sufficient unsaturations are present, a veritable ‘drying oil film’ will be formed, akin to a varnish on wood. This is how linseed oil-based varnishes and paints work. However, this is not desirable in a lubrication situation.
A strategy to increase the oxidative stability of vegetable oils has been the development of high-oleic varieties. These are usually genetically engineered to contain no 18:3 and as little 18:2 as possible. These varieties used to be difficult to source but are now widely available. One can now find high-oleic canola, soybean, sunflower, safflower oils, among others. High oleic oils offer much improved oxidative stability and are the oil of choice in deep fat frying operations, which usually operate at ~180oC.
Another important strategy to minimise or slow down oxidation is the addition of antioxidants. Antioxidants will react with fatty acid free radicals, donate a hydrogen radical and thus terminate the fatty acid oxidation reaction, but become free radicals themselves. They then undergo termination reactions with free radicals of similar stabilities, ie, other antioxidant-free radicals, thus not contributing to the propagation of the reaction. Phenolic antioxidants are some of the most effective and inexpensive antioxidants around. There are many natural alternatives, such as tocopherols or catechins, but they are not nearly as effective at synthetic ones, such as tert-butyl hydroxyquinone (TBHQ), butylated hydroxyanisole (BHA), propyl gallate (PG) and pyrogallol (PY).3
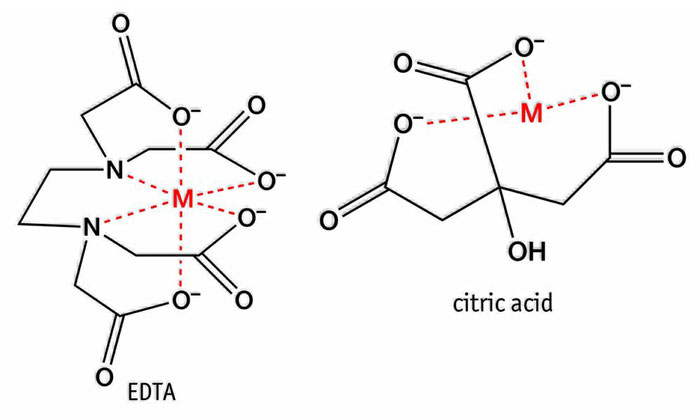
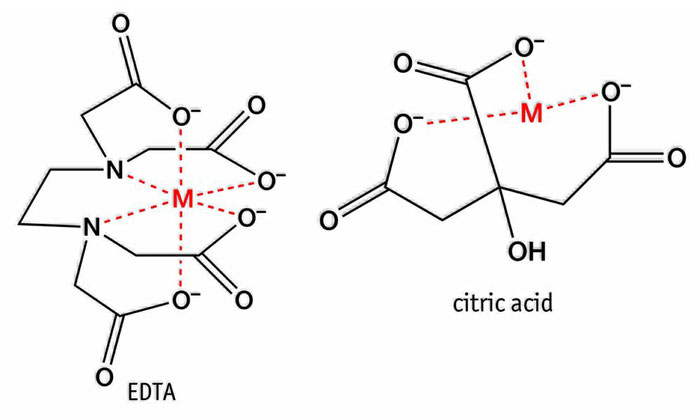
Figure 4: Structures of effective chelators – citric acid and EDTA
One example of the power of antioxidants is illustrated in Table 2. A standard method used to assess the resilience of oils against oxidation is the Rancimat method. Here, air is bubbled through oil held at 110oC. The air is then passed through water and the conductivity of that water is continuously monitored. The onset for the increase in conductivity is reported at the induction time of oxidation. The conductivity increases when secondary oxidation products are formed, such as acids, aldehydes, alcohols and others. Note that soybean oil only has about eight hours of life under those conditions. Upon addition of 100, 200 and 400ppm of TBHQ, the induction time of nucleation increases to almost 40 hours. High oleic oils fare better.4
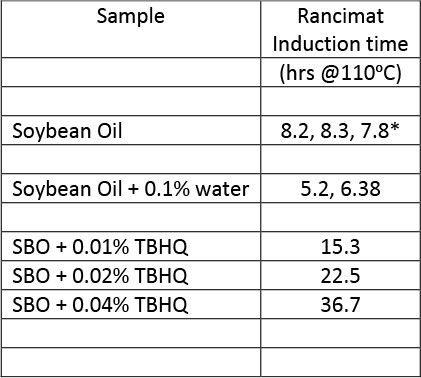
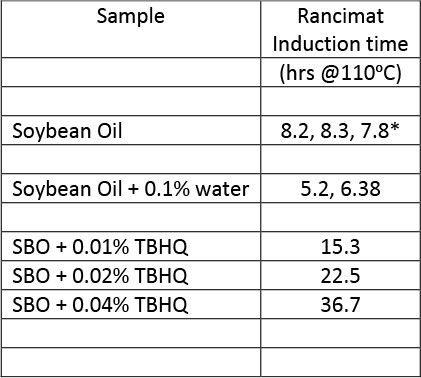
Table 2. Induction time oxidation of soybean oil at 110oC, with and without added tert-butylated hydroxyquinone (TBHQ) antioxidant.
The initiation step of the auto-oxidation reaction can also be affected by several other factors. Ultraviolet light enhances the initial free radical formation, as well as pigments (chlorophyll) and transition metals, particularly iron (Fe+2) and copper (Cu+). This is problematic since a lot of iron is present in lubrication environments. One important strategy to prevent these catalysts wreaking havoc with the oil is to chelate them. This effectively removes them from the reaction mix. Effective chelators include citric acid and ethylenediamine tetraacetic acid (EDTA). These structures are shown in Figure 4.
However, it is important to note that it is not chemically possible to achieve the same oxidative stabilities as saturated mineral oils (Table 1). The chemistry is just not there. Thus, the degraded biobased lubricant must be changed more often.
Conclusion
In this short review, we have introduced the very first change that needs to take place when converting a petroleum-based lubricant (oil, grease, hydraulic fluid) into a biobased lubricant: replacing mineral oil with vegetable oil. The main limitation of vegetable oils discussed here is that of oxidative/thermal stability, which is very important. We have highlighted the mechanisms of degradation of common fatty acids in oils and compared them to mineral oils. We have also highlighted strategies that can be used to stabilise such vegetable oils. Issues such as crystallisation of oils at low temperatures, VI, lubricity, and seal compatibility, will be subjects of a future article.
About the author
Alejandro G. Marangoni, PhD, FRSC works at Whistler Performance Lubricants Inc. in Canada.
References
- Geueke, B. 2017. Dossier – Mineral Oil Hydrocarbons. Food Packaging Forum. DOI: 10.5281/zenodo.820984 (https://www.foodpackagingforum.org/food-packaging-health/mineral-oil-hydrocarbons)
- Wenthold PG. Homolytic C-H Bond Dissociation Energies of Organic Molecules. LibreTexts (https://chem.libretexts.org/Bookshelves/Organic_Chemistry/Supplemental_Modules_(Organic_Chemistry)/Fundamentals/Homolytic_C-H_Bond_Dissociation_Energies_of_Organic_Molecules). Accessed March 27, 2021.
- de Guzman R, Tang H, Salley S, Ng SKY. 2009. Synergistic Effects of Antioxidants on the Oxidative Stability of Soybean Oil- and Poultry Fat-Based Biodiesel. J. Amer. Oil Chem. Soc. 86:459–467.
- Merrill LI, Pike OA, Ogden LV, Dunn ML. 2008. Oxidative stability of conventional and high-oleic oils with added antioxidants. J. Amer. Oil Chem. Soc. 85:771-776.
Related topics
Fats & oils, Natural, Research & development, Technology & Innovation