The aerosolisation phenomenon
- Like
- Digg
- Del
- Tumblr
- VKontakte
- Buffer
- Love This
- Odnoklassniki
- Meneame
- Blogger
- Amazon
- Yahoo Mail
- Gmail
- AOL
- Newsvine
- HackerNews
- Evernote
- MySpace
- Mail.ru
- Viadeo
- Line
- Comments
- Yummly
- SMS
- Viber
- Telegram
- Subscribe
- Skype
- Facebook Messenger
- Kakao
- LiveJournal
- Yammer
- Edgar
- Fintel
- Mix
- Instapaper
- Copy Link
Posted: 20 August 2020 | Christine Faille, Erwan Billet | No comments yet
Aerosol droplets and cross-contamination risks in the food industry. By Christine Faille and Erwan Billet.
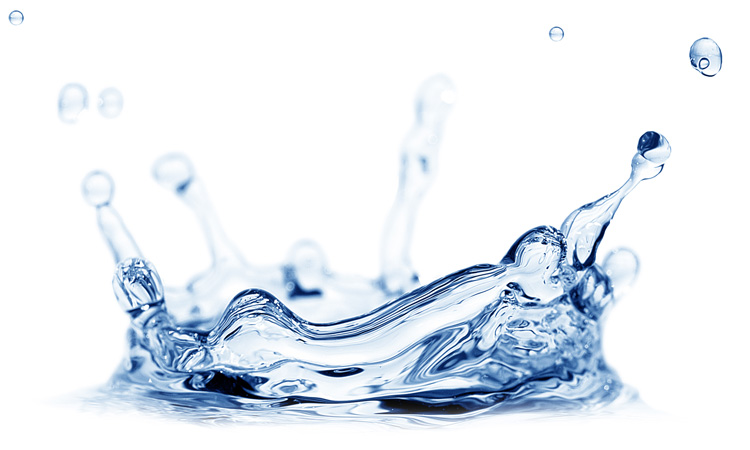
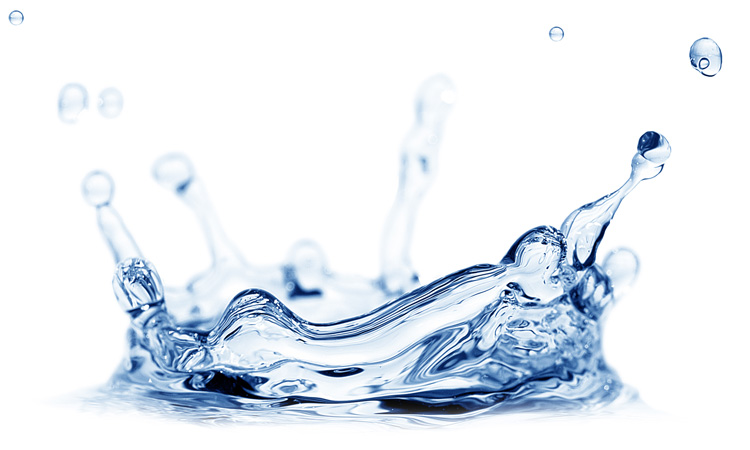
Industrial food contact surfaces are now widely recognised as a main source of food contamination. Surfaces can become contaminated through direct food contact, but also via splashes or aerosol droplets (bio-aerosols) that contain food or sanitation product residues, as well as bacteria, moulds, yeasts or viruses.
According to the World Health Organization (WHO), aerosol transport occurs in droplet form when >5 μm, and in airborne form when ≤5 μm. This article focuses on contamination transmission by droplets.
Washing raw poultry under running water provides a good illustration of this aerosolisation phenomenon. This common practice often results in splashing and potential pathogen transmission (eg, Campylobacter jejuni, Salmonella, Clostridium perfringens) to nearby kitchen areas, with water droplets capable of traveling more than 50cm in every direction.
During food processing, aerosols can be dispersed by air or water flow and originate from a variety of sources. One prime example is ventilation systems; droplets containing microorganisms can be dispersed from condensate on the cooling fins of evaporative chillers.1 Viable aerosols are also often a by-product of cleaning or rinsing, the flooding or cleaning of drains is a frequent source of aerosol formation and of spoilage and pathogenic bacteria, including Listeria monocytogenes. These aerosols would also increase airborne microflora for around 40 minutes.2
Newly formed aerosols could either be directly projected onto surfaces or dispersed from high to low-pressure on air currents within the processing area
High-pressure (40-60 psi) cleaning also generates aerosols and promotes migration of L. monocytogenes from contaminated drains to food-surfaces or food.3
Other surfaces which could contribute towards cross-contamination include floor tiles. Holah et al. (1990) demonstrated the potential for Listeria to be spread around food production areas by a high‑pressure/low volume spray lance, or a low‑pressure/high-volume hose. This practice favours aerosol formation as it forces air out of the drain as the liquid enters.
Aerosols can also form during processing, for example during salad-washing in ‘jacuzzi-style’ systems, where air bubbles bursting on the wash water surface generate droplet aerosols containing microorganisms.4
Transport of aerosols
Newly formed aerosols could be either directly projected onto surfaces or dispersed from high to low-pressure on air currents within the processing area. As aerosols can remain suspended in the air for various periods, they can contaminate new areas at different distances from the droplet formation.
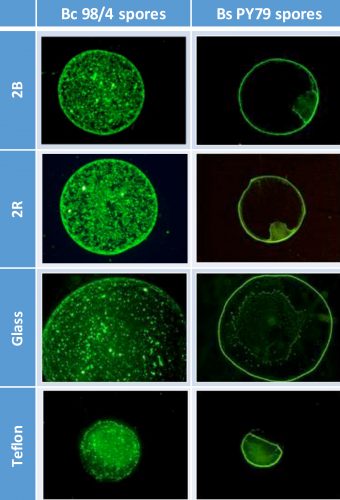
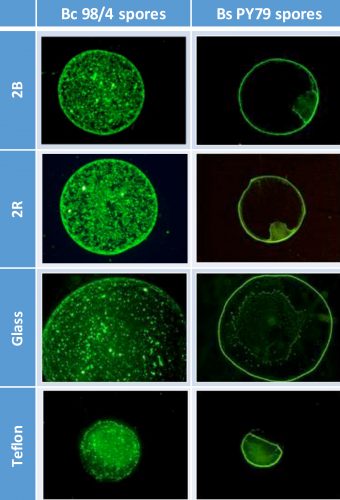
Figure 1: Deposition patterns of Bacillus spores (Bc 98/4 and Bs PY79) on different materials,
examined by epifluorescence (x50)
Not only is transport and ultimate settling affected by the size, density and shape of the aerosol particles,5 but also by environmental parameters such as air currents, humidity and temperature.6 For example, boot washer brushes can disperse quite large droplets one to two meters from source,1 while other floor-washing procedures can disperse these droplets to distances sometimes exceeding two meters in height and five meters in length from the point of impact.7 It has also been shown that hand‑washing produces aerosols detectable more than 10 meters away.8
The main issue is that some microorganisms are able to survive within droplets. For example, L. monocytogenes can live up to 210 minutes in aerosol suspensions.9 It would appear that biphasic decay occurs within droplets in the first one to two minutes, resulting in a rapid loss of viability, followed by a second phase characterised by a slow decrease. It has been suggested that the initial decrease is related to evaporative cooling and mass transfer processes within the droplets until equilibrium is reached.10
Elsewhere, the bacterial survival is affected by the droplet size; as shown on Pseudomonas syringae and Erwinia herbicola, viability loss decreased.11 As mentioned earlier, it is also highly likely that many microorganisms within the droplets will be injured due to the stress of aerosolisation and, therefore, may not form any colonies on culture media, making them very difficult to detect.12
Even in the absence of water, some pathogens have been proven to survive in the air when associated with dust particles.13 Consequently, in the presence of aerosols, it is probable that new surfaces will be contaminated with viable, if not cultivable, microorganisms.
Role of surface properties on the droplet evaporation and deposition patterns
Materials contaminated by bio-aerosol can have varying surface properties, whose physicochemical and topographic differences impact the ease of contamination control. When a sessile droplet containing insoluble micro-particles evaporates, various deposition patterns are observable. The final deposit pattern is the result of the fluid flow within evaporating droplets, which is affected by numerous parameters, such as the properties of the substratum and the particle, the suspending medium, and the external environment. It can be assumed that the structure of the final deposit would affect the further bacterial survival and resistance to cleaning and disinfection procedures.
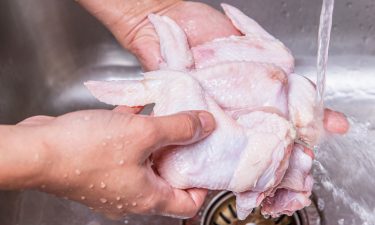
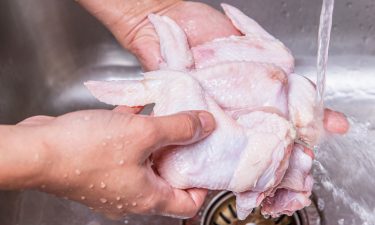
Washing chicken often results in splashes, with droplets capable of travelling more than 50cm in every direction
Few articles have addressed these issues in the context of bacterial contamination in the food environment. The only parameters still to be investigated in this context are substratum wettability14 and topography.15 At INRAE-PIHM, as part of a French National Research Agency (ANR, FEFS project [ANR-18-CE21-0010]) project, we investigated the effects of material surface properties on the deposition of droplets contaminated with Bacillus spores. We first confirmed the main role of material hydrophobicity on the diameter and pattern of the deposit after droplet (1 µl) evaporation (Figure 2). The deposit was small (+/- 1 mm diameter) on a hydrophobic material (Teflon), much larger (2.5-3.5 mm diameter) on hydrophilic glass, and intermediate on stainless steels 316 with a 2B- or 2R-finish. Furthermore, a ring surrounding the whole deposit was observed, whatever the material. More surprisingly, marked differences in the deposition patterns were observed with hydrophilic (B. subtilis) vs hydrophobic (B. cereus) spores, whether in the size of the deposit (larger with hydrophobic spores), or in the distribution of the spores within the ring. When hydrophilic spores were used, the inner area was unevenly covered with small zones covered with numerous spores, the remaining area being poorly contaminated. Conversely, with hydrophobic spores, homogeneous distribution of spores or spore clusters covered the whole inner area.
We also investigated the possible role of material topography through the use of stainless steels with different finishes. Figure 1 shows the surface average roughness, ranging from 0.05 µm or less (glass, polypropylene, stainless steel 2R) to 0.23 µm (stainless steel 2B), seemed to have little or no effect on the distribution of spores over the contaminated zone.
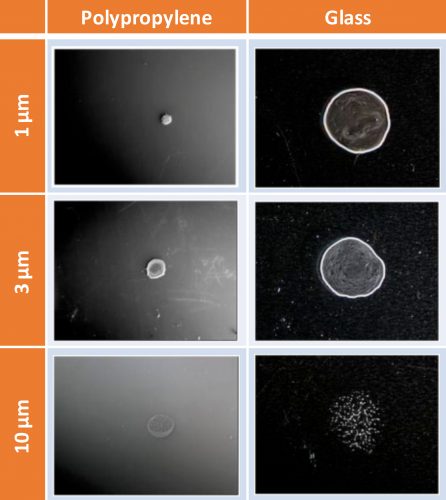
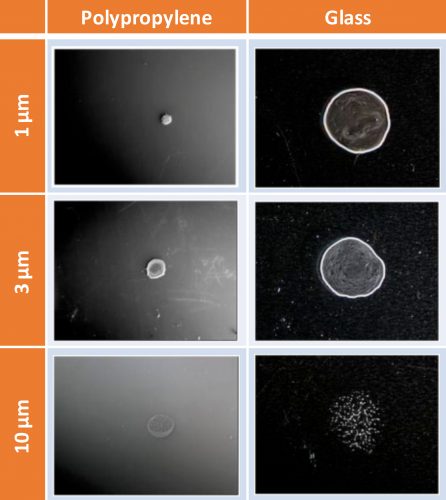
Figure 2: Deposition patterns of fluorescent microspheres (1 µm diameter) on different materials, examined by epifluorescence (x50)
We then investigated the possible role of temperature and particle size on the deposition patterns. In food environments, contaminants can range in size from less than 1 µm (bacteria) to more than 10 µm (yeast), and the temperature varies depending on the process. Initially, we were unable to highlight any significant differences in the deposition patterns of either Bacillus spores as a function of temperature, within the 10-30°C range (data not shown). In order to determine any particle-size influence, we used differing diameter polystyrene microspheres (1, 3 and 10 µm). As shown in Figure 2, the microsphere distribution over the surface is clearly affected by particle size, the main differences being observed between microspheres of 3-µm and 10-µm diameters. This suggests that striking droplet behavioural differences exist for bacteria or yeasts, with likely consequences in terms of survival from desiccation and/or resistance to cleaning and disinfection.
Surface hygiene consequences
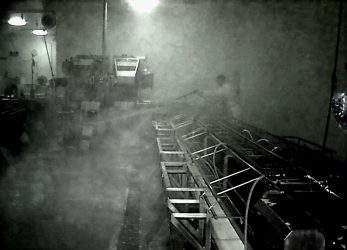
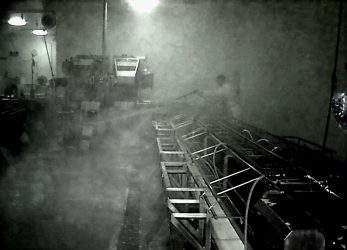
Floor washing procedures can disperse contaminated droplets across long distances
It is worth noting that these newly contaminated surfaces are probably rarely cleaned promptly after contamination. Unfortunately, many bacteria are able to withstand periods of desiccation, whether in the form of biofilms,16 or that of adherent cells17 and, therefore, will remain capable of cross‑contaminating food. One may wonder whether this drying phase might influence the further cleaning resistance of bacteria. Literature on the drying of adherent microorganisms mainly focuses on cross-contamination between foods and surfaces.18
However, at INRAE-PIHM, we have recently shown that the duration of an evaporation step, as well as the temperature during this step, could greatly affect the ease of removal of adherent Bacillus spores.19
Acknowledgements
The authors would like to acknowledge the ANR (Agence Nationale de la Recherche) for funding the FEFS project (ANR-18-CE21-0010) and the Hauts-de-France region for funding the Interreg Veg-I-Tec project (programme Interreg V France-Wallonia-Flanders, GoToS3NuTeX).
References
1. Brown KL, Wray S. Control of airborne contamination in food processing [Internet]. Hygiene in Food Processing: Principles and Practice: Second Edition. Woodhead Publishing Limited; 2013. 174–202 p. Available from: http://dx.doi.org/10.1533/9780857098634.2.174
2. Kang YJ, Frank JF. Characteristics of Biological Aerosols in Dairy Processing Plants. J Dairy Sci [Internet]. 1990;73(3):621–6. Available from: http://dx.doi.org/10.3168/jds.S0022-0302(90)78712-7
3. Saini K, Marsden L, Fung J, Crozier-Dodson A. Evaluation of Potential for Translocation of Listeria monocytogenes from Floor Drains to Food Contact Surfaces in the Surrounding Environment Using Listeria innocua as a Surrogate. Adv Microbiol. 2012;02(04):565–70.
4. Sawyer B, Elenbogen G, Rao KC, O’Brien P, Zenz DR, Lue-Hing C. Bacterial aerosol emission rates from municipal wastewater aeration tanks. Appl Environ Microbiol. 1993;59(10):3183–6.
5. Brandl H, Fricker-Feer C, Ziegler D, Mandal J, Stephan R, Lehner A. Distribution and identification of culturable airborne microorganisms in a Swiss milk processing facility. J Dairy Sci. 2014;97(1):240–6.
6. Chao HJ, Schwartz J, Milton DK, Burge HA. Populations and determinants of airborne fungi in large office buildings. Environ Health Perspect. 2002;110(8):777–82.
7. Holah J. Cleaning and Disinfection Objectives. Ref Modul Food Sci [Internet]. 2018;1–7. Available from: http://dx.doi.org/10.1016/B978-0-08-100596-5.21203-1
8. Burfoot T. Aerosols as a contamination risk. In: Lelieveld H, Holah J, Gabric D, editors. Handbook of Hygiene Control in the Food Industry. 2nd ed. Woodhead Publishing, Elsevier; 2016. p. 81–7.
9. Spurlock AT, Zottola EA. Growth and attachment of Listeria monocytogenes to cast iron. J Food Prot. 1991;54(12):925–9.
10. Fernandez MO, Thomas RJ, Garton NJ, Hudson A, Haddrell A, Reid JP. Assessing the airborne survival of bacteria in populations of aerosol droplets with a novel technology. J R Soc Interface. 2019;16(150).
11. Lighthart B, Shaffer BT. Increased airborne bacterial survival as a function of particle content and size. Aerosol Sci Technol. 1997;27(3):439–46.
12. Kang Y-J, Frank JF. Biological Aerosols: A Review of Airborne Contamination and its Measurement in Dairy Processing Plants. J Food Prot. 1989;52(7):512–24.
13. Mullane NR, Whyte P, Wall PG, Quinn T, Fanning S. Application of pulsed-field gel electrophoresis to characterise and trace the prevalence of Enterobacter sakazakii in an infant formula processing facility. Int J Food Microbiol. 2007;116(1):73–81.
14. Baughman KF, Maier RM, Norris TA, Beam BM, Mudalige A, Pemberton JE, et al. Evaporative deposition patterns of bacteria from a sessile drop: Effect of changes in surface wettability due to exposure to a laboratory atmosphere. Langmuir. 2010;26(10):7293–8.
15. Susarrey-Arce A, Marin A, Massey A, Oknianska A, Díaz-Fernandez Y, Hernández-Sánchez JF, et al. Pattern Formation by Staphylococcus epidermidis via Droplet Evaporation on Micropillars Arrays at a Surface. Langmuir. 2016;32(28):7159–69.
16. Rodríguez A, McLandsborough LA. Evaluation of the transfer of Listeria monocytogenes from stainless steel and high-density polyethylene to Bologna and American cheese. J Food Prot. 2007;70(3):600–6.
17. Kusumaningrum HD, Riboldi G, Hazeleger WC, Beumer RR. Survival of foodborne pathogens on stainless steel surfaces and cross-contamination to foods. Int J Food Microbiol. 2003;85(3):227–36.
18. Jensen DA, Friedrich LM, Harris LJ, Danyluk MD, Schaffner DW. Quantifying transfer rates of Salmonella and Escherichia coli O157:H7 between fresh-cut produce and common kitchen surfaces. J Food Prot. 2013;76(9):1530–8.
19. Faille C, Bihi I, Ronse A, Ronse G, Baudoin M, Zoueshtiagh F. Increased resistance to detachment of adherent microspheres and Bacillus spores subjected to a drying step. Colloids Surfaces B Biointerfaces. 2016; 143:293–300.
About the authors
Issue
Related topics
Related organisations
French National Research Agency, World Health Organization (WHO)