The application of seafood processing by-products in the food industry
- Like
- Digg
- Del
- Tumblr
- VKontakte
- Buffer
- Love This
- Odnoklassniki
- Meneame
- Blogger
- Amazon
- Yahoo Mail
- Gmail
- AOL
- Newsvine
- HackerNews
- Evernote
- MySpace
- Mail.ru
- Viadeo
- Line
- Comments
- Yummly
- SMS
- Viber
- Telegram
- Subscribe
- Skype
- Facebook Messenger
- Kakao
- LiveJournal
- Yammer
- Edgar
- Fintel
- Mix
- Instapaper
- Copy Link
Posted: 18 August 2016 | Dr. Reza Tahergorabi, Assistant Professor, Food and Nutritional Sciences, NC A&T State University (USA) | No comments yet
It has been reported by numerous popular media that fish stocks are declining and several commercial fisheries are currently over-exploited and will collapse by the mid-century. On the other hand, as with other foods, seafood processing generates large quantities of by-products. A typical example is fish filleting to recover boneless and skinless marketable fillets. The frames, heads, and viscera would be typical by-products and the fillets would be considered the main product. When fish are processed for fillets, the by-products account for 60–70% and fish meat and oil left on the by-products account for 20–30% and 5–15% of the whole fish weight, respectively.
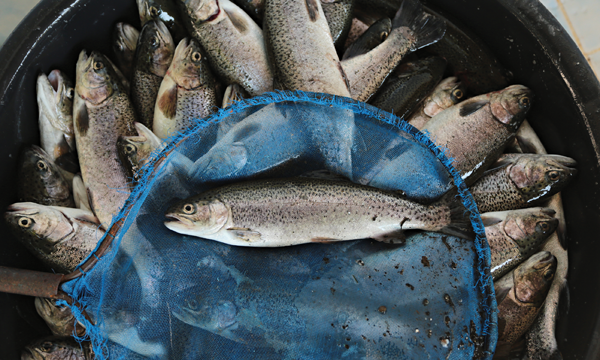
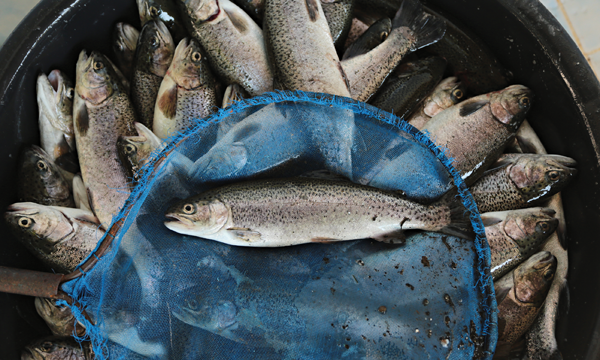
These by-products are nutrient rich but are often ground and discarded without any effort for nutrient recovery. Normally these materials are used as plant fertilisers or livestock feeds, which are considered as a low value-addition. However, recovery and conversion of the by-products to human food, or specialty food, would result in a higher value-addition and this is estimated to increase fivefold within the next decade. Therefore, an appropriate recovery method of seafood processing by-products can result in added revenue for a processor, as well as reduce environmental pollution due to disposal of the processing by-products.
Fish muscle proteins can be recovered from the by-products through a relatively novel processing technology called isoelectric solubilisation/precipitation (ISP)1 . This technology relies on the isoelectric point (pI) of muscle proteins. The pI of a protein is the pH at which the net (i.e., overall) electrostatic charge of the protein is zero. This means that the pI value can affect the solubility of protein at a given pH.
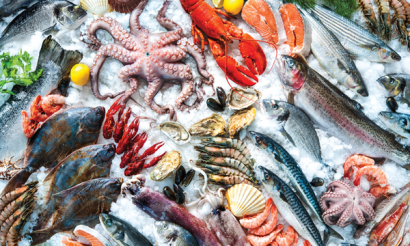
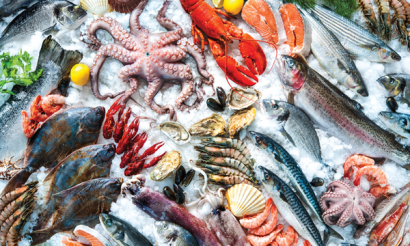
Seafood processing generates large quantities of by-products
There are five steps to the recovery of proteins and lipids from seafood processing by-products using ISP. In the first step, by-products are homogenised in water at a ratio of 1:6 (by-products: water, wt/wt). The aim is to provide a reaction medium and increase the available surface area for the subsequent protein solubilisation reaction.
In the second step the muscle protein is dissolved by the addition of an acid or base to the solution. This Incorporation of acid or base, moves the pH away from the pI – therefore, fish muscle proteins assume a more negative or positive surface charge for alkaline or acidic conditions, respectively. This leads to protein– protein electrostatic repulsion, which weakens the protein-protein hydrophobic interactions with a simultaneous increase of protein–water electrostatic interactions. Ultimately, these changes lead to protein solubility in water.
In the third step centrifugation separates the solution for light, medium and heavy fractions containing fish oil, dissolved muscle proteins and impurities (bones, scale, skin, insoluble proteins, etc.), respectively. Thereby, the third step results in separation of crude seafood oil that is rich in ω–3 polyunsaturated fatty acid (PUFAs) which can be further processed for numerous food and nonfood applications. It is difficult to remove the membrane phospholipids due to their amphiphilic nature. More than 50% of the membrane phospholipids are retained with the dissolved proteins after third step. Normally, membrane phospholipids are present in smaller amounts in the seafood muscle than triglycerides. However, the membrane phospholipids contribute more to rancidity and lipid oxidation. Therefore, it is desirable to remove as much lipid as possible during the separation step. In contrast, the hydrophobic triglycerides are fairly easy to separate from the solution. The heavy fraction is rich in minerals such as Ca, Mg, and P; and can therefore be a main ingredient in the development of animal feeds and value-added pet foods2 .
In the fourth step the medium fraction, containing dissolved seafood muscle proteins, is subjected to the second pH adjustment, or the pI. The average pI of the seafood muscle proteins is 5.50. At 5.50pH, muscle proteins precipitate due to increased protein–protein hydrophobic interactions and decreased protein–water interactions, as well as decreased protein–protein electrostatic repulsion. In a similar way to the first pH adjustment in the second step, as the proteins gradually stop interacting with water the viscosity increases significantly. This viscosity issue can be overcome by continuous maintenance of the pH at 5.50. In the fifth step the precipitated seafood muscle proteins are separated from the process water, typically by centrifugation. The process water can then be recycled and used in the first step if a continuous system is used3 .
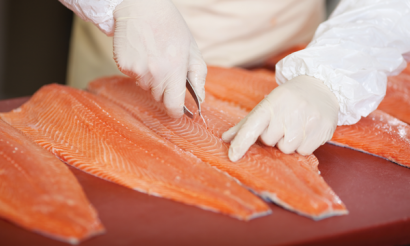
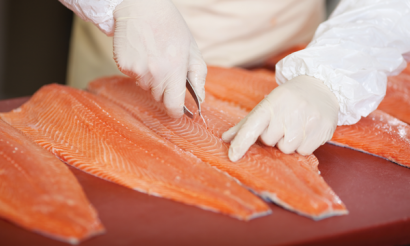
Fish fillet by-products account for 60-70% of the whole fish weight
ISP protein with high functionality has been isolated from various aquatic species at bench-top and pilot-plant levels in batch systems4,5. Chen et al. (2007)1 proposed and tested an ISP system working in a continuous mode of operation to isolate functional protein from krill, whole fish, and seafood processing by-products. However, few researchers have attempted to develop food products from the recovered proteins. Tahergorabi et al., (2011)6 indicated that ISP allows the recovery of proteins from skin-on bone-in dark chicken-meat processing by-products without removal of bones, skin, and fat prior to processing. The addition of TiO2 to proteins recovered from these by-products allows development of heat-set gels with colour and texture comparable to chicken breast gels.
Furthermore, an attempt was made to develop a functional food product that would resemble respective products made from boneless skinless chicken breast meat. A threeprong strategy to address diet-driven cardio – vascular disease (CVD) with a functional food was used in this study. The strategy included the addition of three ingredients with well-documented cardiovascular benefits: (i) ω-3 PUFA-rich oil (flaxseed-algae, 9: 1); (ii) soluble fibre; and (iii) salt substitute. Titanium dioxide, potato starch, polyphosphate, and transglutaminase were also added. The batters were formulated and cooked resulting in heat-set gels. This study demonstrated a feasibility to develop functional food made of muscle proteins recovered with ISP from low-value dark chicken meat processing by- products. The functional food developed in this study had comparable instrumental quality attributes to respective products made of chicken breast meat7.
Fish protein isolate (FPI) was also recovered from whole gutted trout using ISP. FPI was used as a main ingredient in heat-set gels made with ω-3 PUFAs oils (flaxseed, algae, fish, krill, and blend) and KCl-based salt substitute. This study showed that the functional food products developed from FPI were nutritionally enhanced with ω-3 PUFAs. The ω -6/ ω -3 FAs and unsaturated/saturated FAs ratios and the indices of thrombogenicity and atherogenicity, depended on specific ω-3 PUFAs-rich oil used to fortify protein isolate gels. Lipid oxidation in ω-3 PUFAs fortified gels was minimal, although greater (P <0.05) than with control gels (without ω-3 PUFAs fortification). The developed food products had reduced sodium and increased potassium; while the colour and texture properties were good and gelation properties were improved7-9.
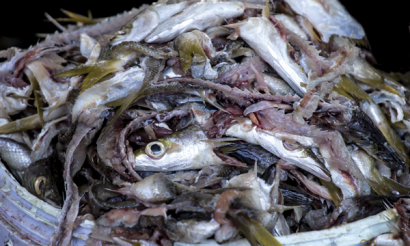
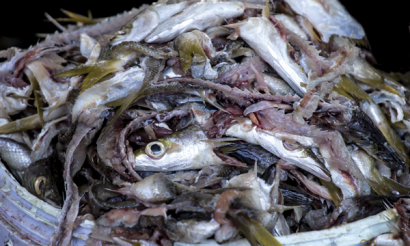
By-products are nutrient rich but often discarded
Normally, NaOH and HCl are used to dissolve and precipitate fish muscle proteins in ISP, therefore contributing to increased Na content in the recovered fish protein isolates (FPI). Substitution of NaOH with KOH may decrease the Na content in FPI and, thus, allow development of reduced-Na seafood products. Recently, FPI was recovered with ISP using NaOH or KOH. In order to develop a nutraceutical seafood product, the FPI was extracted with NaCl or KCl-based salt substitute and subjected to cold- or heat-gelation. In addition, standard nutraceutical additives (ω-3 fatty acids-rich oil and dietary fibre) along with titanium dioxide (TiO2) were added to FPI. A reduction (P <0.05) of Na content and simultaneous increase (P <0.05) in K content of FPI gels was achieved by the substitution of NaOH with KOH during ISP and NaCl with the KClbased salt substitute during formulation of the FPI paste. Although cooking and the addition of NaCl during formulation of the FPI paste increased (P <0.05) lipid oxidation in FPI gels, TBARS values were far below rancidity levels. These results indicate that KOH can replace NaOH to recover FPI from whole gutted fish for subsequent development of nutraceutical seafood products tailored for the reduction of diet-driven cardiovascular disease9.
These studies have shown that the muscle proteins recovered with ISP retain their gel-forming ability and, therefore, can be used as a functional and major ingredient in human food products such as surimi seafood (commonly referred to as crab-flavoured seafood). However, for a commercial success, it will be necessary to develop final applications for the recovered materials. A similar model to the dairy and soybean processing industries could be used for the development of final food products. Government agencies as well as academia should be actively engaged in the collaborative development of final food products.
References
- Gehring, C. K., Gigliotti, J. C., Moritz, J. S., Tou, J. C., & Jaczynski, J. (2011). Functional and nutritional characteristics of proteins and lipids recovered by isoelectric processing of fish byproducts and low-value fish: a review. Food Chemistry, 124(2), 422-431
- Chen, Y. C., & Jaczynski, J. (2007). Protein recovery from rainbow trout (Oncorhynchus mykiss) processing by-products via isoelectric solubilisation/precipitation and its gelation properties as affected by functional additives. Journal of Agricultural and Food Chemistry, 55(22), 9079-9088
- Torres, J. A., Chen, Y. C., Rodrigo-Garcia, J., Jaczynski, J., & Shahidi, F. (2007). Recovery of by-products from seafood processing streams. Maximising the Value of Marine By-products, 65-90
- Mireles DeWitt, C. A., Nabors, R. L., & Kleinholz, C. W. (2007). Pilot plant scale production of protein from catfish treated by acid solubilization/isoelectric precipitation. Journal of Food Science, 72(6), E351-E355
- Nolsøe, H., & Undeland, I. (2009). The acid and alkaline solubilization process for the isolation of muscle proteins: state of the art. Food and Bioprocess Technology, 2(1), 1-27
- Tahergorabi, R., Beamer, S. K., Matak, K. E., & Jaczynski, J. (2012c). Isoelectric solubilization/precipitation as a means to recover protein isolate from striped bass (Morone saxatilis) and its physicochemical properties in a nutraceutical seafood product. Journal of Agricultural and Food Chemistry, 60(23), 5979-5987
- Tahergorabi, R., Beamer, S. K., Matak, K. E., & Jaczynski, J. (2013). Chemical properties of ω-3 fortified gels made of protein isolate recovered with isoelectric solubilisation/ precipitation from whole fish. Food Chemistry, 139(1), 777-785
- Tahergorabi, R., Sivanandan, L., Beamer, S. K., Matak, K. E., & Jaczynski, J. (2012a). A three-prong strategy to develop functional food using protein isolates recovered from chicken processing by-products with isoelectric solubilization/precipitation. Journal of the Science of Food and Agriculture, 92(12), 2534-2542
- Tahergorabi, R., Beamer, S. K., Matak, K. E., & Jaczynski, J. (2012b). Functional food products made from fish protein isolate recovered with isoelectric solubilization/precipitation. LWT-Food Science and Technology, 48(1), 89-95 10. Tahergorabi, R., Beamer, S. K., Matak, K. E., & Jaczynski, J. (2011). Effect of isoelectric solubilization/precipitation and titanium dioxide on whitening and texture of proteins recovered from dark chick
- Tahergorabi, R., Beamer, S. K., Matak, K. E., & Jaczynski, J. (2011). Effect of isoelectric solubilization/precipitation and titanium dioxide on whitening and texture of proteins recovered from dark chick