Partially hydrogenated oils: A blessing and a curse
- Like
- Digg
- Del
- Tumblr
- VKontakte
- Buffer
- Love This
- Odnoklassniki
- Meneame
- Blogger
- Amazon
- Yahoo Mail
- Gmail
- AOL
- Newsvine
- HackerNews
- Evernote
- MySpace
- Mail.ru
- Viadeo
- Line
- Comments
- Yummly
- SMS
- Viber
- Telegram
- Subscribe
- Skype
- Facebook Messenger
- Kakao
- LiveJournal
- Yammer
- Edgar
- Fintel
- Mix
- Instapaper
- Copy Link
Posted: 23 December 2014 | Chad Gray, R&D Food Technologist – Lipids Division, Kerry | No comments yet
A new food era was born in America on 8 November 2013, when the Food and Drug Administration (FDA) made a tentative announcement that the presence of partially hydrogenated oils (PHOs) in food and beverage offerings will no longer be ‘generally recognised as safe’.
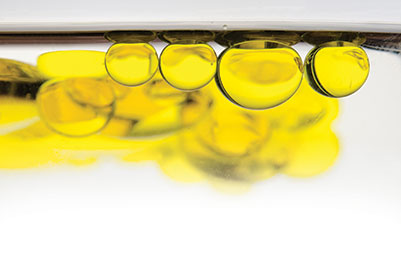
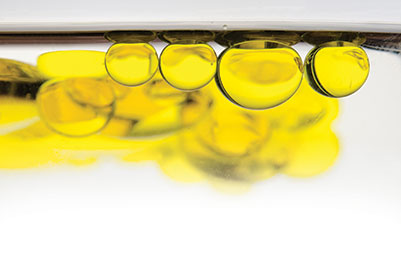
These oils contribute trans fat to the diet, which is linked to an increased risk of coronary heart disease. To reduce their consumption, it is proposed that the PHOs will need to be approved as additives before being allowed for release onto the market. Though the FDA has not definitively lowered the gavel for removal of these ingredients, the demand for ‘product cleansing’ by food manufacturing companies has been felt in shockwaves across the nation, and resonates within the heart of the consumer body. In anticipation, research and development teams everywhere are shifting their focus to removing PHOs from their labels. We see it in data records of 2014, where 10 per cent of new product launches in food contained a low/no/reduced trans fat claim – up from 7.9 per cent in 2013. For some, the switch is simple. For others, it is a nightmare, as it presents several complex technical challenges regarding shelf life, flavour and mouth-feel.
Hydrogenation was introduced to the world in the early 1900s as a means of supporting the dwindling supply and growing demand for beef tallow and butterfat. These high melt-point fat sources possessed the capability to maintain structure and nutritional value under processing conditions, in addition to imparting unique textures to products. Finding a supplemental fat source that could perform well in all of these areas was yet to be found, and certainly couldn’t be expected from the lower melt-point oils that were in plentiful supply.
With hydrogenation, however, low melt-point oils were transformed to reach higher melt-points, becoming harder and more stable to oxidative degradation. Whale and fish oils – which are extremely susceptible to oxidation – were made consumable and affordable due to this process. Soybean oil was transformed into a butterfat alternative. A variety of hydrogenated vegetable oils became the basis for margarines. Further enthusiasm erupted because these products out-performed the traditional fats by spreading at cold temperatures3. In time, the spray drying process was used to create emulsifier systems. Use of hydrogenated oils in this application can mean doubling the shelf life from six to 12 months. Shelf lives of products are determined by their shortest shelf-life ingredient. Emulsifiers serve as a critical functional component to many food systems, so the impact here is immense.
How are PHOs responsible for these functional successes? What are the technical challenges in PHO replacement? To answer these questions, we must first develop an understanding of what hydrogenation is, what it does to the structure of the oil, and the effects this has on key product characteristics.
Hydrogenation can be performed on a lipid* that contains at least one double bond. Double bonds which organise the chain in the cis configuration cause the chain to jut outward (see Figure 1, structure B). These chains are difficult to stack tightly with one another, so the molecules can flow – resulting in a softer, lower melt-point lipid. The saturated and trans structures (Figure 1, structures A and C, respectively) are linear, forming tightly-packed chains and thus a firmer fat with a higher melt-point.
During the hydrogenation process, hydrogen is introduced in the presence of a catalyst to remove the double bonds – turning structure C into structure A. If a chain has more than one double bond and the reaction is not carried out to remove all of them, the lipid is only partially hydrogenated.
At this stage, it seems contradictory that the FDA is calling PHOs out for being the most significant source of trans-fat in processed foods1. The hydrogenation process – complete or partial – is based on removing double bonds, and thus cis and trans configurations. Unfortunately, this is not what exclusively happens. When a double bond is removed, the carbon chain is allowed to spin into the more thermodynamically-favoured linear position. A side reaction involves the removal of hydrogen from the chain; reinstalling a double bond and creating a trans fat when originally it was oriented as cis4.
The FDA is also trying to control trans fat from a usage perspective. Across an individual’s diet, a variety of foods are consumed. If only a few, yet popular food classifications have high levels of trans fat, intake can remain too high1. This makes it all the more critical that applications which use PHOs out of convenience to achieve desired effects, invest great efforts in overcoming the technical challenges for their replacement.
Good nutrition has always been essential, but only lately is it gaining hold as a major focus in the U.S. market. Consumers are seeking better-for-you options, and are more attentive to the ingredient declarations. The public is vocalising their concern in various social media tools. In the past 90 days, more than 65,000 comments on trans fats and PHOs have been created. Furthermore, a Mintel survey reports that 44 per cent of adult women want to know as much as possible about the ingredients in a product before they buy it. This includes knowing about PHOs.
Consumers should know that fat is not something to exclude, but to choose wisely and moderate. While many topics around different types of oils and their health benefits are controversial, the avoidance of trans fats is not5. This topic is the downfall of PHOs. While the FDA itself advises to keep trans fat consumption “as low as possible”, the World Health Organization advises less than one per cent of energy intake1,6.
While trans fat consumption has a negative impact on health, so does consuming less stable lipids that have been degraded. A common method for a lipid to degrade is by reacting with oxygen. Double bonds are very reactive sites – especially for oxygen – and are most plentiful in low melt-point polyunsaturated oils (cottonseed, corn, soybean, etc.). Reaction with oxygen produces a variety of compounds including free radicals, free fatty acids, aldehydes, and so on. These compounds are representative of a decreased nutritional value, and are associated with negatively pungent aromas and tastes. Fewer double bonds to host the reaction imply an increased oxidative stability.
Oxidative stability fosters great taste and flavour delivery, which are essential to providing a quality product. The majority of flavours are oil-soluble, thus lipids help to retain higher concentrations of flavours during processing. Higher solid fats at application and body temperatures tend to support added flavours more so than thinner oils, so partially hydrogenated varieties excel here.
Flavours come from a few areas: additive flavours, the taste of the oils themselves, and the other ingredients in the system. All sources are tightly interrelated. The other ingredients in the system may be masked or accentuated by the fat sources themselves. A clear example of this is coffee and creamer. If the creamer is based on coconut oil, ‘dairy-like’ and ‘sweet’ sensory observations are made, with great ability to block the bitterness and acidity from the coffee. The same creamer formulation, save a swap for sunflower oil, will mask different areas of the coffee profile and come across as rather ‘green’, ‘plantlike’, and even ‘nutty’. Bitterness may be slightly more pronounced in this version as well.
No matter how impressive a flavour is on its own, its expression in the end-product may be disastrous if the texture is not appropriate to support and release it. The proper lipid choice is able to take control of the consistency due to its deceptively simplistic ability to be liquid or solid at very specific – or indeed very unspecific – temperatures. The hydrogenation process allows customisation of this melt-point or range by increasing and shifting it to some desired level. The change imparts a unique texture or mouth-feel that’s otherwise difficult to achieve by using other ingredients. Consumers have come to expect specific textures from their favourite products. Without securing this trait, R&D teams can (and very much do) find themselves developing in circles.
A good-bodied mouth-feel in beverages is close to that of whole milk – one that is distinctively thicker than water and remains on the tongue only for a short period of time after swallowing. Such texture is connected with both indulgence and quality. However, establishing a rich mouth-feel does not mean rushing to grab the fat with the highest melt-point. In fact, you may lose mouth-feel, or generate one with an undesirably long-clinging, oily coating on the tongue instead.
Constructing the ideal mouth-feel is based on choosing an appropriate melting profile. Lipids that are liquid at room temperature will of course be more consistent throughout tasting. Lipids that undergo phase transition along temperature variations are far more complicated. R&D teams that inspect the melting profiles when they’re formulating are already at an advantage.
Melting profiles are found via various techniques, but the bottom line is the same: per cent solid fat is found at a given temperature and repeated over a span of temperatures from cold to hot. Higher per cent solids are found at colder temperatures. The solid fat content (SFC) is one such method for determining the melting profile. The standard method to find the SFC (per ISO, AOCS, and IUPAC) uses nuclear magnetic resonance (NMR). There are direct and indirect methodologies; the direct method providing more consistent results7.
The direct method uses the free induction decay (FID), acquired after a very short radio frequency pulse is applied to a sample situated in a strong, uniform magnetic field. In principle, the first point of the returning signal is representative of both the solid and liquid phases (Figure 2, Point T). Solid signals decay extremely quickly compared to liquid signals, so the liquid-only portion can be read at Point L as quickly as 70 microseconds after the pulse. Using both data points in the following equation allows for the finding of the SFC at the temperature at which the sample has been conditioned:
The equation has a modification to allow for the fact that the sample probe ‘rings’ for a few microseconds after the pulse is applied. Sensible data cannot be recorded during this ‘dead time’. This is a problem only for Point T, as the solid signal decays rapidly. Thus, the first measurable signal, T*, is acquired 11 microseconds after the pulse. To correct for this, known SFC standard samples are measured, and an empirical factor, f, is determined to correct for the drop in the solid signal7:
Having found the shape of the melting curve, it must be interpreted. Focus on the range between the application temperature and body temperature. The application itself determines what is needed in this range. Figure 3 demonstrates a few melting profiles by SFC.
The best chocolates are made using only cocoa butter as a fat source. The finished chocolate rests at room temperature, where the SFC is about 75 per cent (very high). When held in your hands for a short period of time, the slight increase in temperature of the chocolate is not enough to melt it significantly: the SFC is relatively flat in this region. When consumed, the perception is that of high quality:
- Great body and mouth-feel is perceived at the start of the melt via high SFC
- The melt-point of the cocoa butter is very sharp via the steep SFC slope; the chocolate melts quickly
- No undesirable oily coating is left, providing a ‘clean finish’ due to the zero per cent SFC prior to reaching body temperature.
Cocoa substitutes are sought for a variety of reasons; a few being because cocoa butter can be rather expensive or in relatively low supply against high demand. Figure 3 shows a fractionated palm kernel and palm oil blend that attempts to mimic the cocoa butter melting profile. Indeed it’s quite close, but still not the same. Chocolate made with this blend may feel a little harder in the mouth at the start (higher initial SFC), melt a little faster (the mouth-feel doesn’t feel as thick throughout the majority of consumption with the lower SFC), and leave a slight oily coating on the tongue via the trailing SFC beyond body temperature. These effects are minimised because substitutes are used at very low levels based on regulatory standards and the desire for keeping cocoa percentage claims8.
Cold or hot applications are more complex due to the broader range of temperatures that the product is experiencing. A cold beverage may be iced or refrigerated (0-4°C). Although the common SFC tests go down to only 10°C, general patterns are typically the same at these marginally colder temperatures. Consider the types of coconut in Figure 3. It’s implied that the non-hydrogenated version will not have a higher SFC than the hydrogenated version at 0°C or 4°C. For much closer comparisons however, the additional test is recommended.
Unlike the chocolate example, the consumer doesn’t keep an iced latte (containing a partially hydrogenated (PH) coconut oil creamer, for example) in their mouth long enough to change the entire product’s temperature to body temperature. Instead, the lipid content in the beverage’s external layer contacting the tongue and roof of the mouth is responsible for the perceived mouth-feel. Perhaps swallowing occurs when the thin layer on the tongue reaches 20 or 25°C. More mouth-feel is felt by the PH versus non-PH coconut oil via the higher SFC in this temperature range. Post-swallow, the layer containing PH coconut oil is continuing to melt on the tongue, while the non-PH coconut is already gone. In this application, the quick disappearance of the non-PH version is not ideal – it lacks indulgence and is thin, like water. It’s crucial that some solid fat provides mouth-feel after swallowing, but also that it melts quickly after swallowing so as not to be too clinging.
Hot beverages have similar requirements to cold ones with regards to the SFC profile. Most lipids are completely liquid at these hot beverage temperatures of 74 to 85°C. To impart mouth-feel, the thin layer in contact with the mouth should begin to initiate a very low SFC just above body temperature. Ideally, when the lipid reaches body temperature, the SFC should not have increased much further, so as to avoid a waxy, clinging mouth-feel. Figure 3 shows that PH soybean and PH coconut oils are ideal for hot applications. Even the cocoa substitute seems to have a place here. To determine what the optimal selection is, the other properties of the oils should be compared.
Given the investigation of stability, nutrition, flavour delivery, and mouth-feel, product developers may seek other methods to obtain high scores in all categories. To circumnavigate the trans-fat pitfall, non-hydrogenated, fractionated, or inter-esterified oils are options – though each classification has their own set of weaknesses. Non-hydrogenated oils have melting profiles with less desirable slopes, making them harder to achieve the proper mouth-feel and texture targets. Their shelf lives are also drastically reduced by comparison. Additives – natural or synthetic – help regain at least some of the life, but add to the length of ingredient statements and unfavourably skew consumer perception of the product.
Fractionation involves heating and cooling an oil to isolate and concentrate pieces of the melting profile. These types are often too sharp to easily accommodate the temperature range between the application and body temperatures. Fractionated varieties are also more expensive9.
Inter-esterified oils are those where fatty acids are moved from one triglyceride molecule to another to adjust the melting profile. These types are very expensive, but are made slightly more economically feasible if partially hydrogenated oils are used as raw materials. Though the PHOs are consumed throughout the process, it’s difficult to say whether their involvement will inspire consumer pushback9.
Wherever a company’s opinion of PHOs falls, the U.S. market push against them is so strong that choice of transition is arguably removed. PHO demand is plummeting, thus pulling them from supplier production lines and forcing product reformulations. Still, dark questions lurk. After the rush to reformulate on the gamble of the FDA’s decision, will they reverse their tentative position? When bakery, sweets, and beverages feel the pressure of either lower shelf lives, ingredient declarations filled with mitigating additives, or higher prices, how will consumers see them then?
References
- US Food and Drug Administration. (2013). Tentative Determination Regarding Partially Hydrogenated Oils; Request for Comments and for Scientific Data and Information
- Mintel GNPD. (2014).
- Fiedler, Martin. (2001). Wilhelm Normann and History of Fats. http://www.dgfett.de/history/normann/nr_fiedler.php
- Sajbidor, Jan. (2006). Handbook of Food Science, Technology, and Engineering. Fat Hydrogenation in Food Processing. Ch. 156. (Ed: Yiu H. Hui)
- Cunningham, Eleese. (2011). Is there Science to support claims for coconut oil?
- Derbyshire, Emma (2012). Trans fats: implications for health. Nursing Standard. 27, 3, p51-56.
- Oxford Instruments. (2009). Determination of Solid Fat Content in Edible Oils and Fats by the Official Direct Method (AOCS Cd 16b-93)
oxford-instruments.com/SFC-Direct-Method-pdf - Nieburg, Oliver. (2014). Price Pressures: Will chocolate makers bite the bullet on cocoa butter alternatives?
com - O’Brien, Richard D. (2006). Handbook of Food Science, Technology, and Engineering. Sanitation and Safety for an Oils Processing Plant. Ch. 199. (Ed: Yiu H. Hui)
* ‘Lipids’ encompass a large network of compounds. To avoid confusion over the classification of oils and fats based on their liquid or solid status, use of ‘lipid’ is intended to reference and ignore the physical state of only these two groups.
About the author
Chad Gray is an R&D Food Technologist in the Lipids Division at Kerry. He devotes his time to studying and designing non-dairy spray dried creamer systems and whipped topping powders. Prior to this role, Chad worked in Quality Assurance to implement an information management system across Kerry’s North American production facilities. He graduated with a Bachelor’s degree in Chemical Engineering from the University at Buffalo in New York