Understanding the present is key for the future in cheese processing
- Like
- Digg
- Del
- Tumblr
- VKontakte
- Buffer
- Love This
- Odnoklassniki
- Meneame
- Blogger
- Amazon
- Yahoo Mail
- Gmail
- AOL
- Newsvine
- HackerNews
- Evernote
- MySpace
- Mail.ru
- Viadeo
- Line
- Comments
- Yummly
- SMS
- Viber
- Telegram
- Subscribe
- Skype
- Facebook Messenger
- Kakao
- LiveJournal
- Yammer
- Edgar
- Fintel
- Mix
- Instapaper
- Copy Link
Posted: 4 January 2012 | Alan F. Wolfschoon-Pombo, Research Principal, Cheese and Dairy Technology, Kraft Foods | No comments yet
The food industry, and within it the dairy industry, is experiencing a noticeable change. Novel processing technologies and sustainability are trendy terms. Also, the following concepts are under the same trend: efficient use of raw materials (increased yield), less waste generation, reduction of product losses during manufacturing and overall food chain, reduced utilisation of cleaning and disinfection agents, less energy and water consumption, distinct product quality, efficient packaging, shelf life extension, etc.
In the opinion of the author of this article, changes are good, provided we do not forget where we come from. In the past, we can find the foundations for the future. A wealth of knowledge was generated over the past 140 years since the establishment of the mass action law of Guldberg and Waage that allows us to understand the equilibrium of chemical reactions and with it basic (dairy technology related) reactions like milk acidification and neutralisation, concepts applied to the dairy practice by the dairy scientists Soxhlet and Henkel around 1884. The work of the early pioneers, like Arrhenius, van’t Hof, Pasteur and many others, allows us to understand the dynamics of the reactions taking place when heating milk, and with it, the basis of food processing and food safety.
The food industry, and within it the dairy industry, is experiencing a noticeable change. Novel processing technologies and sustainability are trendy terms. Also, the following concepts are under the same trend: efficient use of raw materials (increased yield), less waste generation, reduction of product losses during manufacturing and overall food chain, reduced utilisation of cleaning and disinfection agents, less energy and water consumption, distinct product quality, efficient packaging, shelf life extension, etc. In the opinion of the author of this article, changes are good, provided we do not forget where we come from. In the past, we can find the foundations for the future. A wealth of knowledge was generated over the past 140 years since the establishment of the mass action law of Guldberg and Waage that allows us to understand the equilibrium of chemical reactions and with it basic (dairy technology related) reactions like milk acidification and neutralisation, concepts applied to the dairy practice by the dairy scientists Soxhlet and Henkel around 1884. The work of the early pioneers, like Arrhenius, van’t Hof, Pasteur and many others, allows us to understand the dynamics of the reactions taking place when heating milk, and with it, the basis of food processing and food safety.
The food industry, and within it the dairy industry, is experiencing a noticeable change. Novel processing technologies and sustainability are trendy terms. Also, the following concepts are under the same trend: efficient use of raw materials (increased yield), less waste generation, reduction of product losses during manufacturing and overall food chain, reduced utilisation of cleaning and disinfection agents, less energy and water consumption, distinct product quality, efficient packaging, shelf life extension, etc.
In the opinion of the author of this article, changes are good, provided we do not forget where we come from. In the past, we can find the foundations for the future. A wealth of knowledge was generated over the past 140 years since the establishment of the mass action law of Guldberg and Waage that allows us to understand the equilibrium of chemical reactions and with it basic (dairy technology related) reactions like milk acidification and neutralisation, concepts applied to the dairy practice by the dairy scientists Soxhlet and Henkel around 1884. The work of the early pioneers, like Arrhenius, van’t Hof, Pasteur and many others, allows us to understand the dynamics of the reactions taking place when heating milk, and with it, the basis of food processing and food safety.
Like many other foods, milk is composed of proteins, fats, carbohydrates, minerals and water. Protein, a word suggested by Berzelius, is the milk component from which cheese is basically made. No one can tell when cheese was first made; it is assumed that it was a nomad, transporting milk in a leather bag (derived from an animal stomach?) who first saw the curdled milk, i.e. the cheese. This curdling of the milk was due either to an acidification process that took place, or to an enzymatic process from the enzymes in the leather bag, or due to both processes taking place simultaneously. The lactic acid formed from the fermentation of the milk sugar caused the clotting of the major milk protein, casein; the enzymes which act proteolytically clotting the casein. Casein micelles are the components from which cheese is made. The casein micelles are formed in the epithelial cells of the mammary gland from amino acids. Biosynthesis takes place on the ribosomes of the rough endoplasmic reticulum (ER) (see Figure 1). From ER, the proteins are transported through the Golgi apparatus (G), where they are phosphorylated and calcium (Ca) and phosphate (PO4 ) are incorporated. In G vesicles, association and aggregation of the submicelles and complex formation with Ca and phosphate PO4 occurs, and the micelles are discharged in the lumen of the alveolus (see Figure 1)2. About six to eight per cent of the dry weight of casein micelles is composed of various minerals of which Ca phosphate is the largest constituent. Caseins exist as spherical particles (micelles) with about 20 – 300 nanometres (average of 150 nanometres) in diameter, comprising between 20 – 150,000 molecules, and holding more water (3g/g protein) than casein sub-micelles. The submicelles contain different casein molecules (αs1-, αs2-, β- and κ-casein, see Table 1), with certain variability in their ratio1-3. To a large extent, the casein micelles determine the stability of milk products during heating, concentration and holding. This is basically due to the dynamic equilibriums between a) the free casein molecules and submicelles, b) between dissolved and colloidal calcium phosphate and c) between free submicelles and micelles1.
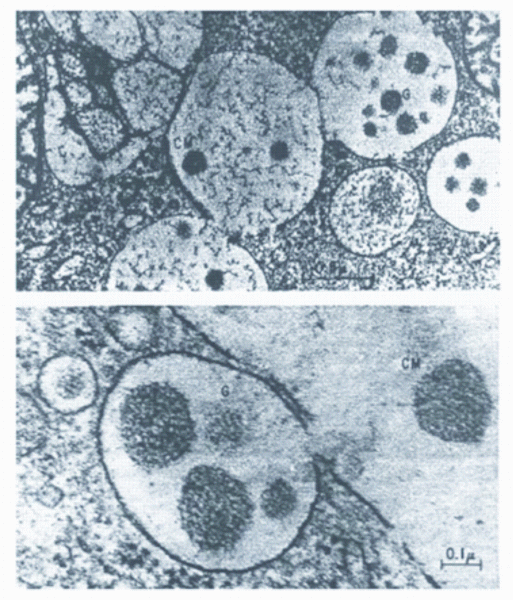
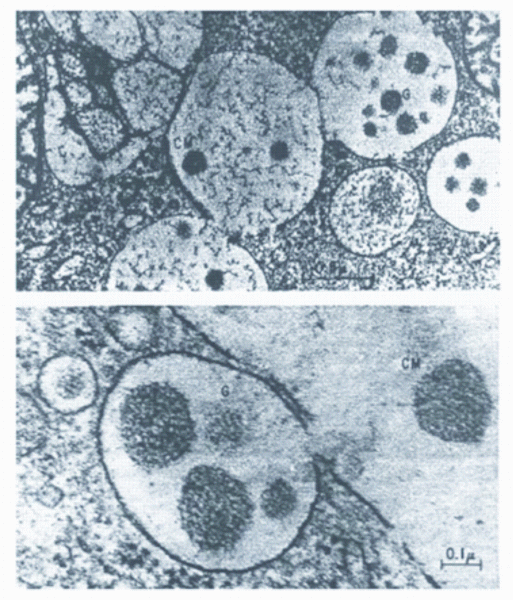
FIGURE 1Formation and discharge of casein micelles into the alveolar lumen of the mammary secretory cells
The milk coagulation is followed by mechanical operations like cutting of the gel and different treatments of the gel curd to remove water and minerals from it, in order to produce cheese with the desired characteristics. Depending on the pressing, salting and curing conditions, cheeses will be consumed very fresh (Quark) to very matured (e.g. Parmesan). Caseins account for about 77 per cent of the total (crude) protein of the milk; 18 per cent are due to the so-called whey proteins and five per cent is the non protein nitrogen fraction. Hereafter, cheese is essentially a concentrated dairy protein gel, a dense network, a matrix in which milk fat globules, whey (with its dissolved solutes), microorganisms and enzymes are occluded or distributed within its pores. The rest is the water phase of the milk, now called whey, containing the whey proteins, some minerals, the rest lactose and some fat. Figure 2 shows many of the parameters influencing cheese composition and quality until the time of the curd separation from the whey, since the amount of whey and minerals retained in the cheese curd determines its basic structure. Cheese is a viscoelastic material. It is evident that whatever the cow is fed also has a direct influence on the cheese made out of this milk.
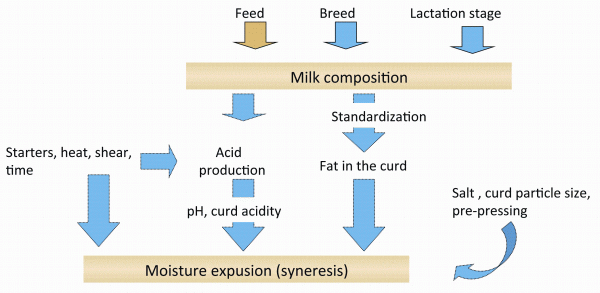
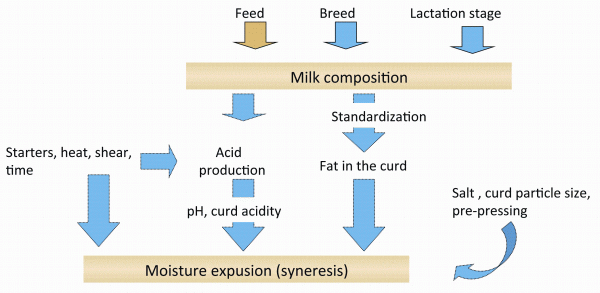
FIGURE 2 Basics aspects of cheese making affecting the water and calcium content and the pH of a rennet gel
Making cheese is an art and also a science. Special cheese vats, devices for whey drainage and curd forming (e.g. Casomatic) and automatic pressing and salting have been developed over the last 70 years. A breakthrough, however, in the way cheese is made was due to the pioneer work of the French scientists Maubois, Mocquot and Vassal, who in the late 1960’s introduced ultrafiltration (UF) as a novel membrane process to separate and concentrate the milk proteins and fat from the whey4. It should be observed that with this process, the totality of the whey proteins – and contrary to traditional cheese making – are concentrated along with the caseins, increasing the yield of the cheese making operation, but yielding different characteristics to the curd (and cheese) made in this form. The UF process is based on the pore size of the membrane, which allows the passage of those milk components which are lower than the pore size, while those bigger in size are retained by the membrane, or simply filtered off. Ultrafiltration has slowly but steadily gained acceptance from the dairy industry, especially for the manufacture of soft cheeses and later for the other types. In this form, the cheese industry has been continuously evolving from handmade to fully automated cheese making.
Approximately 15 years ago, a new membrane processing technique, the micro – filtration (MF), really started to gain acceptance in the global dairy industry. MF, a result of academic research work carried in the late 1980’s by the group of Maubois5 in Rennes, France, is a very versatile membrane processing technology because of the wide range of available pore size, allowing separation and fractionation of fat, casein and whey proteins from the other components. Early adopters have certainly truly benefited in many ways from this technique. Furthermore, MF can be used in combination with other membrane processes – like ultrafiltration (UF), nanofiltration (NF) and reverse osmosis (RO) – to fractionate and concentrate the milk components6-9 and to make cheese and dairy products. Adsorption processes and formation of a primary layer limit flux and protein permeation during protein fractionation with cross-flow microfiltration10. Modification in the ratio of αs-casein to β-casein, standardisation of the protein content of cheese milk, modified mass ratios between the proteins, the minerals and the lactose are approaches that offer possibilities to tailor made functional properties6,7,9. Figure 3 shows schematically the treatment of skim milk by membrane processing for effective protein fractionation11; combining MF and advanced UF process enables the production of different milk protein concentrates.
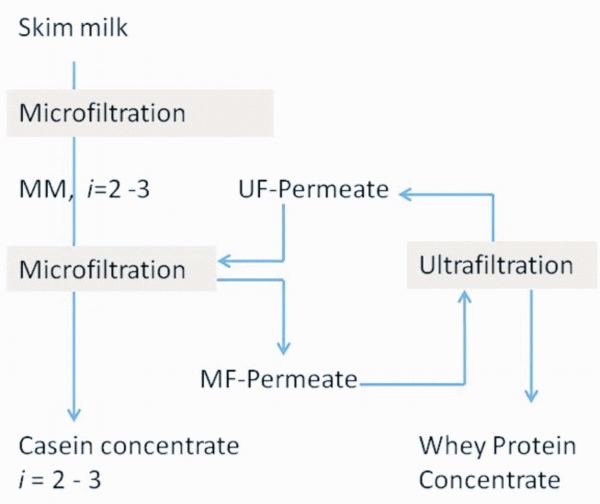
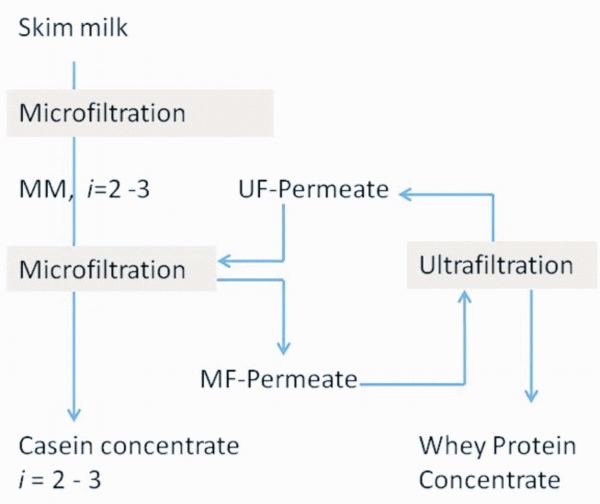
FIGURE 3Scheme of milk protein membrane processing11
One of the MF features is that, based on pore size, it can separate the bacteria from the milk6,7, a treatment normally carried out by the so-called bactofuges (centrifugal separators). A bacteriocidal effect in milk can also be achieved by the use of different time x temperature combinations treatment, which, on its own, results in different microbiological and chemical effects and intensities, and with it, changes to the cheese milk. MF, carried out with 1.4 μm pore size at ~35°-50°C on skim milk, is extremely gentle and effective, and also allows further heat treatment of the retentate (containing the bacteria, cell debris and some heat resistant enzymes) and therefore, different possibilities for its re-utilisation.
The major application of MF in the dairy industry is to selectively separate and concentrate the micellar casein in skim milk, normally using a pore size of ~0.1μm5-7,11. With this casein concentrate, new casein concentrate powders were developed. Also, milk casein enrichment allows the cheese maker to optimise the cheese manufacturing process (yield, quality) and the value of the derived byproducts. Especially in the latter, the filtrate from the microfiltration of skim milk, the so-called ‘ideal whey’ is where a lot of research has been and is being carried out. This fraction contains all the whey proteins, and those milk components soluble in the water phase, in its native state. The whey proteins have been recovered in the form of whey protein UF concentrates from cheese whey, but not in their native state. These concentrates have found applications across the food industry8. The functional properties of the whey proteins, and especially of those found native in the ideal whey, are affected, among other things, by the heat treatment applied to the concentrates.
Recent research work carried out under the group of Professor Kulozik at Weihenstephan in Germany has demonstrated that individual whey proteins recovered from ideal whey have distinct functionalities when micro-particulated. The heat treatment applied in the function – alisation or microparticulation process affects the final functionality of the proteins, because during the heat treatment, the native whey proteins change into a partially reversible molten globule state (partial unfolding) before going into the final denaturated (unfolded state) and aggregated state (irreversible state). The proportions of the proteins in the different states partially dictate the functionality of the concentrate; other factors are for example related to the milieu (pH, minerals, carbohydrate content). Figure 4 shows a diagram12 of time and temperature for ß-Lactoglobulin that leads to the determination of the different proportions just referred to; it shows that for example at 82°C and 38 seconds, 10 per cent of the ß-Lg is irreversibly aggregated, whereas 70 per cent of it is still in the native state and 20 per cent in the unfolded state (so called molten globule state); α denotes the degree of unfolding.
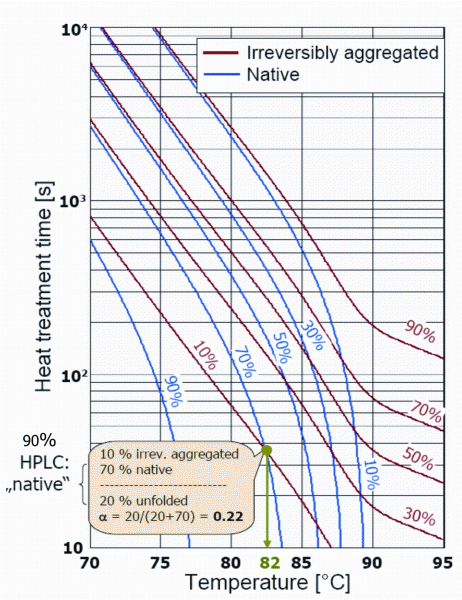
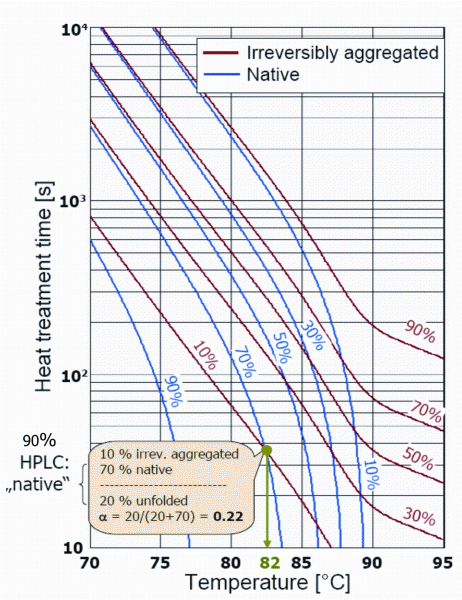
FIGURE 4Temperature-time diagram and denaturation of β-Lactoglobulin12
The concentrated globular (whey) proteins can be included into the structural matrix to produce protein-rich products with defined microstructure and texture. Ingredients properties as well as processing conditions directly influence structure formation. Creating structural elements from the WPC, via additional ingredients and/or pre-texturisation steps, enable changing the texture characteristics in a cheese (as fat does); for example, structural elements prepared from low-protein con – centrate may act as a structural breaker in a high protein matrix13. Conversely, structural elements from high-protein concentrates may act as structural builders in a low- or non-protein matrix13. The size and shape of the particles, as well as the shear applied, play a role in the interactions between themselves and between the structural elements and the continuous phase. The pH at the pre-texturisation (functionalisation) step plays an important role in obtaining round particles or fibrils14. Microparticulation can be achieved by high shear homogenisation, double emulsification or phase separation between biopolymers (protein-polysaccharide)13. With concentrated casein micelles, the use of well-defined shear flow might create new possibilities to induce the formation of new structures; this is due to shear banding (resulting in alignment of the proteins during liquid flow)13,15; contrary to this, and due to particle size and Brownian motion, concentrated globular proteins present a challenge to this new technique.
All the interactions between the proteins in the natural cheese matrix are important. Physical and chemical properties of cheese, such as texture, colour, melt and stretch, are primarily determined by the interaction of casein (CN) molecules16. If we take, for example, the melting of a natural cheese (e.g. Cheddar), we will observe changes and phase separation in concert with the heat applied. From a physical point of view, a substance melts when it is transformed from a ‘solid-like’ into a ‘liquid-like’ state (see Figure 5, page 26). Basically, there are casein interactions via phosphoseryl clusters and (+) charged CCP (colloidal calcium phosphate) and through hydrophobic regions (A in Figure 5). Then, greater thermal motion of the molecules, particles and strands with increasing heating temperature occurs, and with it more rapid relaxation (and breaking) of protein-protein bonds, which facilitates flow (B in Figure 5). The following forces are in play: a) Hydrophobic interactions (attractive) increase with temperature increase (maximum 60°C – 70°C) but a weakening of the gel might result from reduction in contact area between casein molecules; b) Hydrogen bonding (attractive) decreases with temperature increase; c) Electrostatic repulsion increases with temperature increase. Overall, the balance of forces shifts towards more repulsion and weakening of the protein matrix16. In the process of making a processed cheese, the melting process is different to the one as described above, and is here induced by the presence of the so-called melting or emulsifying salts, which chelate calcium from the casein matrix of the natural cheese. This conversion is aided by the heat/shear applied, resulting in re-hydration and solubilisation of the (originally present) insoluble para-calcium caseinate particles from the cheese; the caseinate, now in the form of a soluble para-sodium-phospho-caseinate, allows the formation of the homogeneous cheese mass. The caseinates are manipulated via shearing, heating and pH, to obtain the desired texture. Quoting J.M:Manski15, “the creation of food structures involves deformation to organise and assemble ingredients. In addition, solidification of the structures obtained is required to entrap these structures and to create products with a certain consistency.”
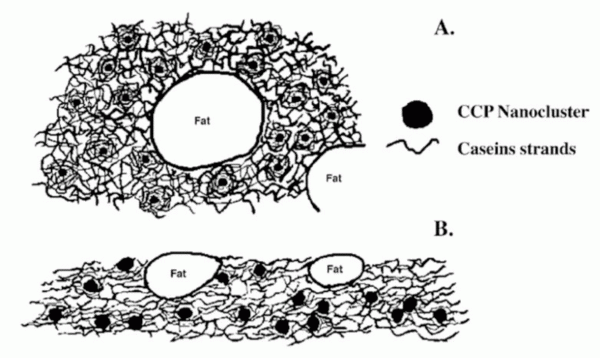
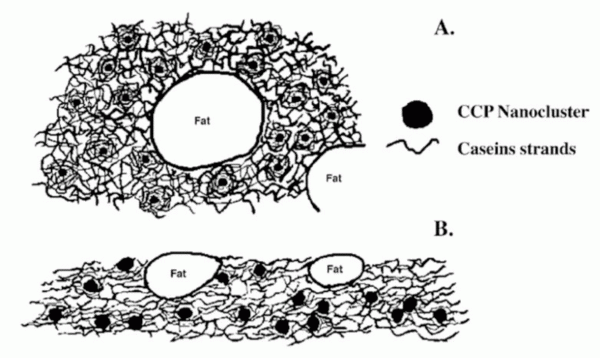
FIGURE 5Schematic drawing of the structure of unmelted(A) and melted (B) natural cheese16
Utilisation of new materials derived from milk fractionation to make dairy products needs to be carefully evaluated and appraised. Many applications and papers have been published6-9, however, each processor has its own formulations to follow (and to develop). Certain questions must be taken into consideration. How do the new ingredients affect the assembly of the cheese structure? What is the effect of simple shear and temperature? How do you control the mineral content to obtain desired textures? How do you control the drag force and to limit the convective transport of protein material that builds a layer on the membrane surface during the fractionation of casein and whey proteins, that eventually lead to low flux and protein permeation values? Basic processes, such as fermentation, coagulation and heating/ shearing, as well as understanding of the membrane process, play a fundamental role in the quality and economics of the dairy product being produced.
The dairy industry is continually trying to be more effective in the utilisation of the raw materials. In future, a strict control of the composition of the dairy components being processed and of the final product(s) will further aid the economics of any new cheese making technology. More automation work in this area will be needed. The food processor and cheese maker will have to harmonise the quality of the product with the capacity and economics of the production process, and to do this, a sound knowledge of the materials being processed, and of the unit operations per se, without overlooking environmental aspects, is required. Only in doing so will the benefits of the new avenues opened by, for example, membrane technologies (milk fractionation) will materialise.
References
1. Walstra, P. & Jenness, R., 1984. Dairy Chemistry and Physics, Chapter 13. Casein Micelles, 229-253, John Wiley & Sons.
2. Farrell, H.M., Jr., Leung, C.T. and Wickham, E.D. 1992. Distribution of ADPase activity in the lactating rat mammary gland and its possible role in an ATP cycle in the Golgi apparatus. Arch. Biochem. Biophys. 292, 368-375
3. P.X. Qi, 2007. Studies of casein micelle structure: the past and the present. Lait,87(4/5)363-383
4. Maubois, J.L., Mocquot, G., Thapon, J.L., Humilier, M-C., Chopin, A., Goudedranche, H., Dupas, C., Blanc-Patin, E., Piot, M., Fauquant, J. (1971). Preparation de fromage á partir de pré-fromage liquid obtenu par ultrafiltration du lait. Lait 508,495-533
5. Fauquant, P., Maubois, J.L.,Pierre, A., 1988. Microfiltration du lait sur membrane minérale. Tech.Lait. 1028, 21-23
6. Maubois, J.L., 2002. Membrane microfiltration: a tool for a new approach in dairy technology. The Australian J. of Dairy Technol. 57(2) 29-96
7. Rosenberg, M., 1995. Current and future applications for membrane processes in the dairy industry. Trends in Food Sci.& Technol. 6,12-19
8. Hinrichs, J., 2008. Neue Verfahren zur Optimierung der Käsereitechnologie. Chem.Ing.Tech. 80(8)1137-1146
9. Mistry, V., Maubois, J.L., (2004) Application of membrane separation technology to cheese production. Cheese, Chemistry, Physics and Microbiology. Third ed., vol.1, 261-285. Elsevier
10. Kühnl, W., Piry, A., Kulozik, U., 2008. Einfluss des Membranwiderstands auf die Filtrationsleistung bei der Proteinfraktionierung entlang von Mikrofiltrationsmodulen. Chem.Ing.Techn. (0(8)1199-1205
11. Kersten, M., 2001. Proteinfraktionierung mittels Membrantrennverfahren. Thesis, TU München, Germany
12. Tolkach, A., 2008. Thermisches Denaturierungs – verhalten von Molkenproteinfraktionen: selektive Denaturierung-Fraktionierung mit Membranen- Reaktions-, Auffaltungs- und Aggregationskinetik. Thesis, Weihenstephan, Germany
13. Purwanti, N., van der Goot, A.J., Boom,R., Vereijken,J., 2010. New directions towards structure formation and stability of protein-rich foods from globular proteins. Trends in Food Sci.& Technol.21,85-94
14. Wolfschoon-Pombo, A., 2010. Microparticulation of whey proteins: Quo vadis? Weihenstephan Technology Seminar, Oct. 29th, TU München, Freising, Germany.
15. Manski, J.M. (2007) Flow-induced structuring of dense protein dispersions. Thesis, Wageningen Universiteit, Netherlands
16. Lucey, J.A., Johnson, M.E., Horne, D.S., 2003. Invited review: perspectives on the basis of the rheology and texture properties of cheese. J.Dairy Science 86:2725-2743
17. Wolfschoon-Pombo, A., 2008, Compreensão do queijo como ingrediente versátil na indústria de alimentos. XXV Congresso Nacional de Laticínios, Jul 14-17th, Juiz de Fora,Minas Gerais,Brasil
Biography
Dr-Ing. Alan F. Wolfschoon Pombo is Research Principal, Cheese and Dairy Technology, at Kraft Foods, based in Munich, Germany. He focuses on dairy research, process and product development and scale up, mainly in natural, fresh and process cheese products. He received a degree in Chemistry from the University of Panamá, a master’s degree in Food Technology from the State University of Campinas, Brazil, and a doctoral degree from the Technical University of Munich, Germany, in 1981. He received a post-doctoral research fellowship from the Alexander von Humboldt Foundation. Alan also worked several years teaching and researching in dairy technology at the Dairy Institute Candido Tostes/EPAMIG, in Brazil.