Making low-fat yoghurts creamier through dynamic pressure processing
- Like
- Digg
- Del
- Tumblr
- VKontakte
- Buffer
- Love This
- Odnoklassniki
- Meneame
- Blogger
- Amazon
- Yahoo Mail
- Gmail
- AOL
- Newsvine
- HackerNews
- Evernote
- MySpace
- Mail.ru
- Viadeo
- Line
- Comments
- Yummly
- SMS
- Viber
- Telegram
- Subscribe
- Skype
- Facebook Messenger
- Kakao
- LiveJournal
- Yammer
- Edgar
- Fintel
- Mix
- Instapaper
- Copy Link
Posted: 4 January 2012 | Mark A.E. Auty, Vivian L. Gee and Christian I. Ciron, Teagasc Food Research Centre | No comments yet
Improving creaminess in food products, whilst simultaneously decreasing fat, remains a challenge for food manufacturers worldwide. Yoghurts are inherently perceived as healthy food products and can be made even healthier by reducing fat. However, reducing fat in yoghurt without compromising desirable textural characteristics like creaminess is difficult to achieve. One approach is to add fat replacers such as modified starch, polysaccharide-based hydrocolloids or microparticulated proteins. Fat replacers or fat mimetics can improve texture but have the disadvantage of requiring labelling as ‘additives’. An alternative approach is to modify the textural attributes of yoghurt through processing. In this article, high quality low-fat natural yoghurts using industry standard formulations were produced using high dynamic pressure (microfluidisation) technology.
The definition of ‘creaminess’ can be used in reference to product appearance, flavour and/or texture. It is a meta-descriptor that is constantly evolving and transforms when used in context with different products and can vary between individuals. It has been defined as “possessing the textural property producing the sensation of the presence of a miscible, thick, smooth liquid in the oral cavity”1. Fat contributes to mouthfeel and flavour and fat particle size affects micro – structural, rheological, and ultimately, the sensory properties of a variety of milk products, including yoghurt.
Improving creaminess in food products, whilst simultaneously decreasing fat, remains a challenge for food manufacturers worldwide. Yoghurts are inherently perceived as healthy food products and can be made even healthier by reducing fat. However, reducing fat in yoghurt without compromising desirable textural characteristics like creaminess is difficult to achieve. One approach is to add fat replacers such as modified starch, polysaccharide-based hydrocolloids or microparticulated proteins. Fat replacers or fat mimetics can improve texture but have the disadvantage of requiring labelling as ‘additives’. An alternative approach is to modify the textural attributes of yoghurt through processing. In this article, high quality low-fat natural yoghurts using industry standard formulations were produced using high dynamic pressure (microfluidisation) technology. The definition of ‘creaminess’ can be used in reference to product appearance, flavour and/or texture. It is a meta-descriptor that is constantly evolving and transforms when used in context with different products and can vary between individuals. It has been defined as “possessing the textural property producing the sensation of the presence of a miscible, thick, smooth liquid in the oral cavity”1. Fat contributes to mouthfeel and flavour and fat particle size affects micro - structural, rheological, and ultimately, the sensory properties of a variety of milk products, including yoghurt.
Improving creaminess in food products, whilst simultaneously decreasing fat, remains a challenge for food manufacturers worldwide. Yoghurts are inherently perceived as healthy food products and can be made even healthier by reducing fat. However, reducing fat in yoghurt without compromising desirable textural characteristics like creaminess is difficult to achieve. One approach is to add fat replacers such as modified starch, polysaccharide-based hydrocolloids or microparticulated proteins. Fat replacers or fat mimetics can improve texture but have the disadvantage of requiring labelling as ‘additives’. An alternative approach is to modify the textural attributes of yoghurt through processing. In this article, high quality low-fat natural yoghurts using industry standard formulations were produced using high dynamic pressure (microfluidisation) technology.
The definition of ‘creaminess’ can be used in reference to product appearance, flavour and/or texture. It is a meta-descriptor that is constantly evolving and transforms when used in context with different products and can vary between individuals. It has been defined as “possessing the textural property producing the sensation of the presence of a miscible, thick, smooth liquid in the oral cavity”1. Fat contributes to mouthfeel and flavour and fat particle size affects micro – structural, rheological, and ultimately, the sensory properties of a variety of milk products, including yoghurt. There are several reviews dedicated to sensory perception of texture, specifically on the role of rheological and frictional properties of foods2, the mechanism of oral processing in relation to lubrication and deposition behaviour of food components3 and the role of friction in sensations of food texture4. A more recent review examines perception, characterisation and prediction of creaminess in dairy products5. These reviews highlighted that creaminess relies heavily on rheology, mainly viscosity particularly for liquid foods, but its perception is also controlled by lubricating properties, composition and microstructure6,7.
High dynamic pressure processing – microfluidisation
Homogenisation of milk is a very common approach to reduce fat globule size and increase emulsion stability and conventional valve homogenisation is the most common technology used by the dairy industry. Microfluidisation is a high dynamic pressure process used primarily by the Pharma sector to produce very fine stable emulsions of relatively small volume, high value pharmaceuticals. However, microfluidisation technology is not widely used by the dairy industry. The disruption mechanism of this technological approach is based on pressurising the fluid streams to induce particle-particle collisions at ultraturbulent conditions inside a proprietary interaction chamber with fixed-geometry micro-channels. Microfluidisation is an ultrahigh shear process that operates at shear rates much greater than those of classical emulsification technologies, such as colloid mills and valve homogenisers. Microfluidiser® processors can attain shear rates of > 10,000,000 s-18, which makes the mechanical emulsification of fluid food and non-food materials into nano/micro-emulsions possible. The maximum flow rate for most versions of a pilot scale Microfluidiser® is 450 mL min-19, while the maximum flow velocity was estimated to be around 400 m s-110. The shear rate, flow rate and flow velocity of the microfluidisation process are determined and controlled by the applied pressure. The resultant effects are greater emulsion stability11,12 due to change in particle size and this may impart desirable textural properties, such as creaminess.
Production of yoghurt
Yoghurt was manufactured in the Biofunctional Food Engineering Facility in Moorepark as follows: recombined milk was prepared from skim milk powder (10 per cent, w/w) and sucrose (two per cent, w/w). The two dry ingredients were dissolved at 60°C in reverse-osmosistreated water using a high-shear rotor/stator batch mixer (Silverson Machines Ltd., UK) and then held overnight in a cold room (~5°C) to allow equilibration and hydration of milk components. The milk samples were then prewarmed to 60°C in a steam jacketed vessel before processing. For low-fat milk, melted anhydrous milk fat or cream was added. The milk samples were heat-treated at 95°C for two minutes in an UHT/HTST Lab Pasteuriser (Electric Model 25HV Hybrid; MicroThermics Inc., U.S.A.) and then homogenised either in a conventional valve homogeniser (GEA Niro-Soavi S.p.A., Italy) or in a Microfluidiser Processor™ (M110-EH model; Microfluidics Corp., U.S.A.) fitted with a Ychamber (Figure 1). Homogenisation was performed in two stages at 20 and 5 MPa for the conventional method and in a one-stage process at pressures from 50 – 150 MPa for microfluidisation. The processed milk samples were inoculated with FD-DVS YFC-471Yo-Flex® starter culture containing Streptococcus thermophilus and Lactobacillus delbrueckii subsp. bulgaricus (0.02 per cent, v/v; Chr. Hansen, Cork, Ireland). Fermentation was carried out in sterilised 5-L stainless steel beakers (h = 210 millimetres; Ø = 180 millimetres) using a large water bath at 43°C until pH reached a value of 4.7 ± 0.1. The yoghurts were stirred five times (up-and-down motion) using a stainless steel plunger (Ø = 152.4 millimetres) with six perforations (Ø = 30.2 millimetres) 38.1 millimetres apart and then cooled to 20°C in a water bath with running chilled water. Stirred yoghurts of approximately 125 grams batch size were apportioned into sterile 200-mL polypropylene conical pots with snap-on caps and kept in a cold room (~5°C) until instrumental testing. Heat-treated (both before and after microfludisation) and non-heat treated milks were also compared. All factors were kept equal except for the type and level of pressure of homogenisation and fat content which ranged from 0.1 per cent to six per cent fat. The quality (water holding capacity, particle size), textural properties and microstructure of the yoghurts were characterised using confocal and electron microscopy, rheology, dynamic light scattering and texture profiling tech – niques13-15. In parallel, the yoghurts were tested by a trained sensory panel.
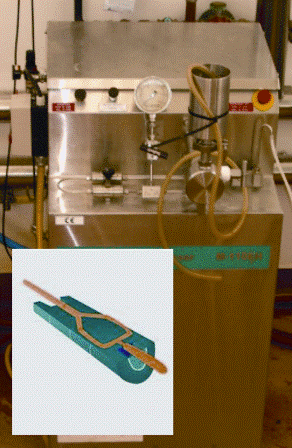
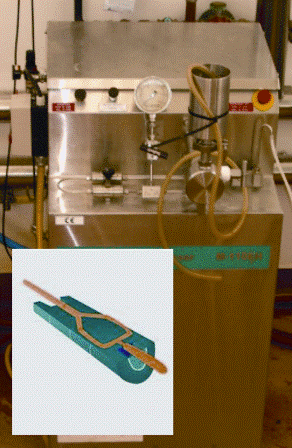
FIGURE 1 The Microfluidics™ high pressure microfluidiser in the Moorepark Biofunctional Food Engineering facility, inset shows the Y-chamber geometry
Sensory studies
Full descriptive sensory data was obtained for yoghurts produced using different fat levels and processing regimes. Thirty-seven identifiable attributes to describe the texture, mouthfeel and creaminess of the yogurt were generated by the trained sensory panel. Samples tested included the yoghurts made as well as commercial samples in a variety of fat levels. The sensory panel was able to distinguish between the yoghurts made using different processing techniques and fat contents. Principal component analysis plots indicated that the low-fat (1.5 per cent) yoghurt processed using MFZ was significantly creamier than standard conventionally homogenised (CH) yoghurt at the same fat content. In fact, the 1.5 per cent MFZ samples were similar in texture and creaminess to a 3.5 per cent CH yoghurt. This advantage was also seen at 3.5 per cent and six per cent fat yoghurts as the 3.5 per cent MFZ was similar to a six per cent CH yoghurt.
Rheological analysis
Rheological analysis of yoghurts indicated that high pressure treatment by MFZ reduced gelation time and produced a firmer gel (Figure 2) and also significantly increased viscosity (Figure 3); a result that was more pronounced at low fat contents. These benefits could translate to benefits to the industry as a reduced holding time results in faster turnover and greater efficiencies in production lines.
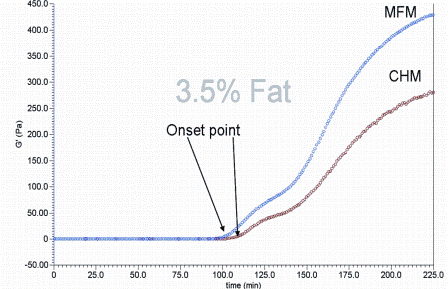
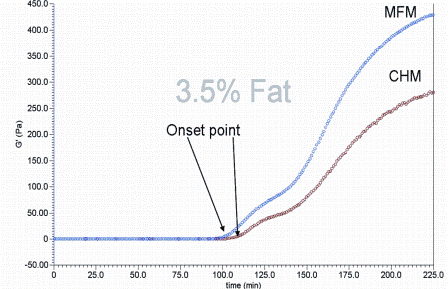
FIGURE 2 Time-course changes of storage modulus (G’) during fermentation of 3.5 per cent fat yoghurt produced with conventionally (CHM) and microfluidised (MFM) milk
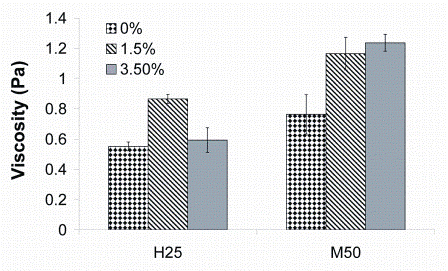
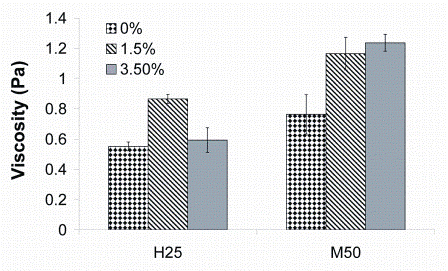
FIGURE 3 Apparent viscosity of yoghurt: effect on of microfluidisation (M50) at 50 MPa compared with conventional homogenization (H25) for different fat contents (0, 1.5 and 3.5 per cent)
How does microfluidisation result in creamier mouthfeel? Microstructure is the key. Analysing the change in microstructure is key to understanding why the yoghurts were superior in terms of creaminess. Yoghurt microstructure was analysed by state-of-the-art imaging equipment at the National Food Imaging Centre. Results from confocal scanning laser microscopy showed that high pressure MFZ treatment created a finer fat/protein matrix (Figure 4) in which a more highly interconnected fat to protein gel network was produced. Cryoscanning electron microscopy, which shows the yoghurt in as close to native form as possible without chemical processing, and Scanning Transmission Electron Microscopy confirmed the change in the structure of the gel network as the fat globule size was reduced significantly (Figure 5). A significant finding was that micro – fluidisation resulted in the production of characteristic fat-protein nano-clusters (Figure 6) that were not present in the conventionally homogenised product. Processing by microfluidisation appeared to physically change the structure by increasing the surface area on the fat globule for interaction with whey and casein micelles or micellar fragments. In addition, the high shear and cavitation forces induced by microfluidisation resulted in a change in the casein-casein and casein-whey interactions which in turn interact within interfacial surfaces on the reduced fat globules upon reassembly. Using advanced microscopic techniques it was confirmed that the samples that were microfluidised exhibited visible differences such as denser protein aggregates and embedded nano-sized (< 100 nanometres) fat globules within protein networks. Viscosity and smoothness are factors relating to perceived creaminess. Increasing the density of the protein matrix relates to increased viscosity and gel strength. It is postulated that a minimum amount of fat (>0.1 per cent and <1.5 per cent) is necessary to produce sufficient fat-protein nano-clusters to mimic the creaminess normally found in full fat products.
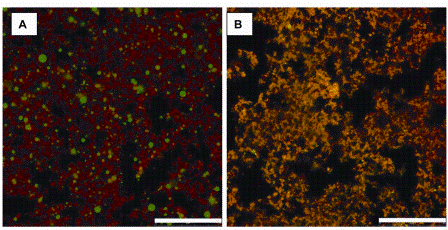
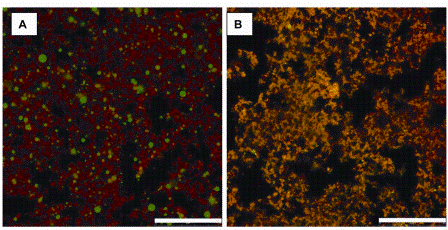
FIGURE 4 CLSM images of 1.5 per cent fat yoghurt made with (A) conventionally homogenised milk and (B) microfluidised milk (50MPa) showing interconnectedness of protein-fat network and the reduction in fat droplet size. Protein is labelled red and fat labelled green. Orange colour of B indicates co-localisation of fat and protein due to subresolution fat droplets. Scale bar = 25μm
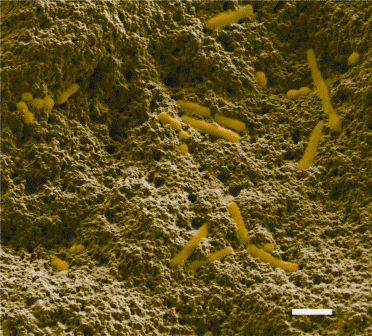
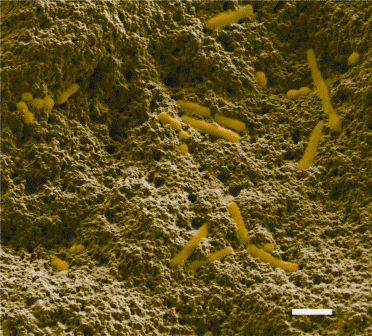
FIGURE 5Cryo-scanning electron micrograph of microfluidised low fat yogurt showing the fine protein/fat matrix with embedded starter culture bacteria: Lactobacillus sp. and Streptococcus sp. (yellow). Scale bar = 1μm
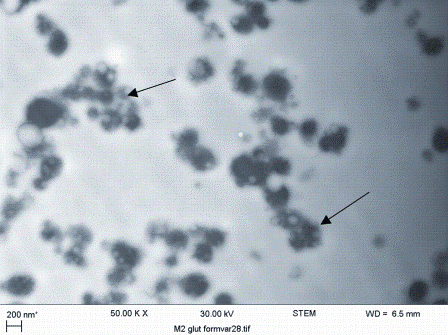
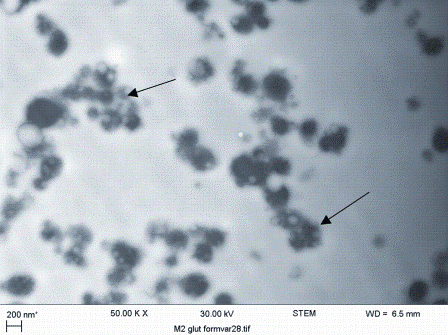
FIGURE 6Transmission electron micrograph of yoghurt made with microfluidised milk showing nano-clusters of fat and protein (arrows)
Summary
Low-fat yoghurts produced from microfluidised milk were as creamy, if not creamier, than their full fat counterparts. This was accompanied by improved water holding capacity, viscosity and texture profiles. Strong relationships were seen between fat content, microstructure, homog – enisation regime and sensory attributes, particularly those contributing to overall creaminess perception. Adoption of this technology would enable the industry to produce low-fat consumer goods with significantly improved creaminess. In-line microfluidisers coupled with conventional HTST equipment are now available and should enable up-scaling to commercial volumes. Low-fat fluid milk, ice creams, frozen dairy desserts and whipped creams could all be produced from microfluidised milks in order to retain the creamy characteristics and low fat credentials demanded by the consumer.
References
1. Jowitt, R. (1974). “The terminology of food texture”. Journal of Texture Studies 5: 351-358
2. Kokini, J. L. (1987). The physical of liquid food texture and texture-taste interactions. Journal of Food Engineering, 6(1), 51-81
3. Malone, M. E., Appelqvist, I. A. M., & Norton, I. T. (2003). Oral behaviour of food hydrocolloids and emulsions. Part 1. Lubrication and deposition considerations. Food Hydrocolloids, 17(6), 763-773
4. De Wijk, R. A., & Prinz, J. F. (2006). Mechanisms underlying the role of friction in oral texture. Journal of Texture Studies, 37(4), 413-427
5. Frost, M. B., & Janhoj, T. (2007). Understanding creaminess. International Dairy Journal, 17(11), 1298-1311
6. Kilcast, D., & Clegg, S. (2002). Sensory perception of creaminess and its relationship with food structure. Food Quality and Preference, 13(7-8), 609-623
7. van Vliet, T., van Aken, G. A., de Jongh, H. H. J., & Hamer, R. J. (2009). Colloidal aspects of texture perception. Adv Colloid Interface Sci, 150(1), 27-40
8. Microfluidics. (2010). Technical Bulletin TB-LV-1 (LV1 Low volume Microfluidizer® processor) Newton, MA, USA: Microfluidics International Corporation
9. Microfluidics. (2008). Technical Bulletin TB-EH-3 (M- 110EH-30 Microfluidizer® processor). Newton, MA, USA: Microfluidics International Corporation
10. Perrier-Cornet, J. M., Marie, P., & Gervais, P. (2005). Comparison of emulsification efficiency of proteinstabilized oil-in-water emulsions using jet, high pressure and colloid mill homogenization. Journal of Food Engineering, 66(2), 211-217
11. Dalgleish D, Tosh, S. M., & West, S. (1996). Beyond homogenization: the formation of very small emulsion droplets during the processing of milk by a Microfluidizer. Netherlands Milk and Dairy Journal 50: 135-148
12. Olson DW, et al. (2004). Effect of pressure and fat content on particle sizes in microfluidized milk. Journal of Dairy Science 87: 3217-3223
13. Ciron, C.I.E, Gee, V.L., Kelly, A.L., Auty, M.A.E. (2010). Comparison of the effects of high-pressure microfluidization and conventional homo genization of milk on particle size, water retention and texture of non-fat and low-fat yoghurts. International Dairy Journal 20: 314 – 320
14. Ciron, C.I.E, Gee, V.L., Kelly, A.L., Auty, M.A.E. (2011). Effect of microfluidization of heat-treated milk on rheological and sensory properties of reduced-fat yoghurt. Food Hydrocolloids 25: 1470-1476
15. Ciron, C.I.E., Gee, V.L., Kelly, A.L. and Auty, M.A.E. (2012). Modifying the microstructure of low-fat yoghurt by microfluidization of milk under different pressures to enhance rheological and sensory properties. Food Chemistry 130: 510 – 519
Biography
Mark A.E. Auty is a Senior Research Officer at the Teagasc Food Research Centre, Moorepark, and is an inter – nationally recognised expert on food microstructure with over 25 years experience in applying microscopy techniques to understanding of food behaviour. He has a BSc in microbiology (Surrey) and PhD in dairy chemistry (UCC, Cork). He has published 39 peer reviewed articles and is a frequent invited speaker at international conferences. His main research interests are food structurefunction relationships, dynamic microscopy techniques and food nanotechnology. In 2007, he commissioned and set up the world’s first publically funded fully integrated imaging centre dedicated to food research, comprising light, confocal, electron and atomic force microscopes.
Vivian L. Gee is a Research Officer in the Food Chemistry & Technology Department at Teagasc Food Research Centre, Moorepark. Vivian received both her Bachelor of Science and Master of Science degrees in Food Science and Technology from the University of Alberta. She has a strong interest in food structure research and has spent the past five years in Teagasc using various imaging, rheological and calorimetric techniques to characterise milk proteins, yoghurts and infant food formulas. Vivian is currently pursuing a PhD with a focus on microscopy and food structure.
Christian ‘‘Ian’’ E. Ciron has a Master’s degree in statistics from the University of the Philippines (Diliman) and has just successfully completed his PhD which is entitled: ‘The Impact of Microfluidization on the Structure, Sensory properties and Creaminess of Yoghurt’ under the co-supervision of Dr Mark Auty and Prof. Alan Kelly (University College Cork, Ireland), and has several peer-reviewed publications. His main research interests include structurefunction relationships and linking sensory attributes to food properties using a multidisciplinary approach of microstructural, rheological and sensory evaluation methods to have a better understanding of behaviour of foods, particularly of dairy products.