Surface energy analysis of food moulding and demoulding
- Like
- Digg
- Del
- Tumblr
- VKontakte
- Buffer
- Love This
- Odnoklassniki
- Meneame
- Blogger
- Amazon
- Yahoo Mail
- Gmail
- AOL
- Newsvine
- HackerNews
- Evernote
- MySpace
- Mail.ru
- Viadeo
- Line
- Comments
- Yummly
- SMS
- Viber
- Telegram
- Subscribe
- Skype
- Facebook Messenger
- Kakao
- LiveJournal
- Yammer
- Edgar
- Fintel
- Mix
- Instapaper
- Copy Link
Posted: 1 June 2009 | Jianshe Chen, Senior Lecturer, School of Food Science and Nutrition, University of Leeds | No comments yet
Moulding and demoulding is a processing operation commonly used in the manufacturing of candy and confectionary products for two main purposes: setting and shaping/forming. During moulding, a food material in the form of either flowable fluid (such as a melted sugar solution, a melted gel, etc) or semi-solid (such as biscuit dough) is poured or pressed into the mould. For a semi-solid food, product shaping and formation into a desired geometry is probably the main purpose.
Moulding and demoulding is a processing operation commonly used in the manufacturing of candy and confectionary products for two main purposes: setting and shaping/forming. During moulding, a food material in the form of either flowable fluid (such as a melted sugar solution, a melted gel, etc) or semi-solid (such as biscuit dough) is poured or pressed into the mould. For a semi-solid food, product shaping and formation into a desired geometry is probably the main purpose.
Moulding and demoulding is a processing operation commonly used in the manufacturing of candy and confectionary products for two main purposes: setting and shaping/forming. During moulding, a food material in the form of either flowable fluid (such as a melted sugar solution, a melted gel, etc) or semi-solid (such as biscuit dough) is poured or pressed into the mould. For a semi-solid food, product shaping and formation into a desired geometry is probably the main purpose.
However, for a fluid food, moulding will involve a phase change (setting) as well as shaping and forming. The fluid food would set or solidify through either cooling or thermal treatments. For example, in the manufacturing of chocolate bars, tempered chocolate of approximately 30°C is poured into the mould and then cooled down to between 15 – 20°C for solidification and crystallisation. The set chocolate is then separated from the mould, usually by gently tapping the mould or by slightly twisting the mould. This process is usually known as demoulding.
Demoulding is essentially the separation of the set food from the mould substrate. A major practical problem of demoulding is the sticking of food to the mould surface, which very often leads to surface defects of the product or even a fractured product. Figure 1 shows an example of leftover after the demoulding of chocolate bars, where fractured chocolate fragments spread over the mould surface, reflecting excessive surface defects of the final product. It is generally accepted that a key factor determining whether a demoulding is a clean separation is the balance of two forces: the cohesive force of the food material and the cross-interface bonding between the food and the mould. As a general rule, if the cohesive force of the food is dominantly higher than the cross-interface bonding, the demoulding should lead to a clean surface separation. If, however, the cross-interface bonding between the food and the mould surface is comparable to the cohesiveness of the food, then surface defects or fracture may become inevitable.
The cohesiveness is a material property of the food, representing its cohesive strength against external destructive forces. A highly cohesive food material normally has strong internal bonding and/or cross-linking which helps to hold food particles together against breaking / fracturing. An enhanced cohesiveness of a food material can be achieved in many different ways. For example, the addition of functional ingredients (such as proteins and polysaccharides as structure-building components), the addition of cross-linking agents (Ca++, enzymes), the addition of filler particles (emulsion droplets or fat droplets) or the alteration of processing conditions (such as an increased temperature for protein denaturation, or a decreased temperature for solidfication), either alone or in combination, could all be effective in increasing cohesiveness. However, since increased cohesiveness means a change of physico-chemical and microstructural properties and, therefore, the textural and sensory perception of the food, manufacturers are very reluctant to adopt this approach and see this as the last resource for an improved demoulding operation. Therefore, to have weakened cross-interface bonding between food and the mould surface has always been the focus of moulding and demoulding research.
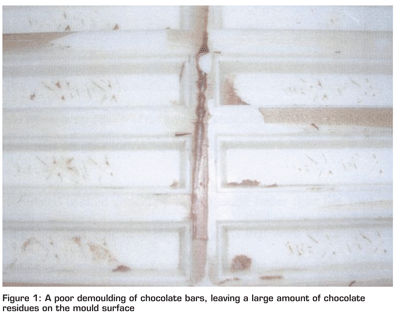
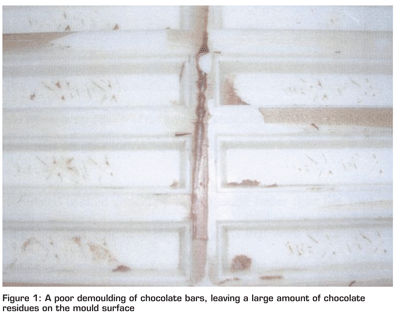
Figure 1


Figure 2
The critical importance of the balance between adhesion and cohesion can be conveniently explained using Figure 2, where a food (A) is in contact with a mould substrate (B). The separation (or demoulding) between the two has two possible scenarios: a neat separation between the two contacting surfaces or a separation causing a breaking through the food material. A preferred separation pathway will naturally be the one which requires less amount of work.
In theory, the total work (Wt) required for demoulding (or surface separation) can be quantified by the total energy of newly created surfaces. For example, for the scenario of a clean demoulding, the work of separation will be the sum of the surface energy of the food and the mould surface (γA×SA + γB×SB), while for a demoulding leading to surface fracture, the work of separation should be equal to the total surface energy of the fractured food surface (2γA×SA), where γ is the surface energy per unit area and S stands for total surface area. Based on this argument, the main guidance for a proper clean surface separation will be simply to use a mould which has a surface energy lower than that of the food (γB < γA). And for this reason, glass is a bad choice as a mould material not only because of the safety concern, but also because of its very high surface energy. Stainless steel has relatively lower surface energy than glass and is often used in food industry for moulding. The most commonly used materials for food moulding are polymeric materials, such as polycarbonate and Teflon because of their unique advantage of low surface energy.
Surface energy, or surface free energy, is caused by the imbalanced molecular interactions in the surface layer. Such imbalance leads to an excess surface force intending to bring molecules at the surface inwards in order to minimise the surface exposure. A practical implication of surface energy is the ease of spreading or ‘wetting’ of a fluid against a surface. For a liquid material, surface energy is commonly replaced by the term of surface tension which can now be easily and reliably determined using a tensiometer. In contrast to liquids, the surface molecules of a solid material are immobile and can only vibrate around their mean position. Therefore, a solid surface has a non-equilibrium surface structure and is not practically possible to have a direct measurement of its surface energy.
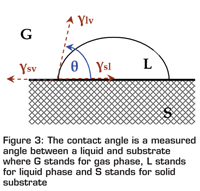
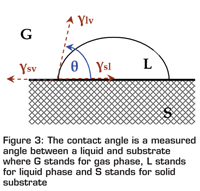
Figure 3
Even so, there have been numerous efforts in trying to develop reliable methods of either indirectly measuring or estimating the surface energy of solid materials. One common approach is to measure the surface contact angles of a number of liquids of known surface tension on the solid surface. This method is based on the principle that the contact angle of a liquid on a solid surface is the net result of the free energy of three contacting surfaces (solid-vapour, solid-liquid and liquid-vapour) (see Figure 3), explained by the well-known Young’s equation:
γsv = γsl + γlv cosθ
where γsv is the solid-vapour interfacial tension, γlv is the liquid-vapour interfacial tension, γsl is the solid-liquid interfacial tension, and θ is the equilibrium contact angle. A low surface tension liquid/fluid will be much easier spreading out on a high energy surface. Examples of contact angle measurements are shown in Figure 4, where three fluids show decreased contact angle (or increased capability of spreading) on a substrate surface due to their decreased surface tension. Based on this principle, one may conclude that a low surface energy mould material and a high surface energy (surface tension) food could be hugely beneficial for moulding and demoulding. For both cases, the fluid will have a poor spreading (wetting) and cross-interface interactions will be much weaker.
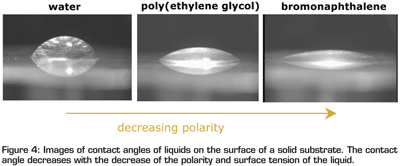
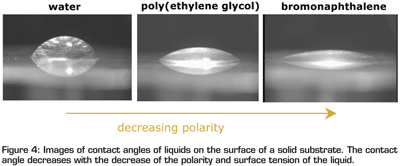
Figure 4
One effective method to estimate the surface energy of a solid substrate is the Zisman’s approach, which measures the contact angle of a number of liquids with known surface tension on the solid substrate. The measured contact angle is then plotted against the surface tension to obtain a linear relationship as shown in Figure 5. A critical surface tension (γc) can be obtained by extrapolating the linear relationship to cosθ = 1 (the point of complete wetting with a zero degree contact angle). This critical value is used to represent the surface energy of the substrate. Many other methods have also been proposed for surface energy characterisation of solid substrates. However, it must be noted that each methodology has its own limits, however accurately it has been designed. It is therefore very important that, for the purpose of comparing and ranking, the same methodology should be used to determine the surface energy of mould materials.
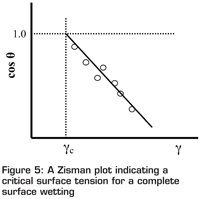
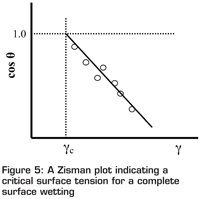
Figure 5
Unfortunately, there has so far been no effective method to determine the surface energy of fluid food materials. Most fluid foods are either too viscous for a correct surface tension measurement using current technologies or too ‘dissolvable’ that any liquid for contact angle measurement will ‘dissolve’ or ‘disappear’ quickly once it is in contact. However, it is possible to have an estimation of surface energy (tension) of some fluids foods. The fluid is diluted continuously so that their surface tension can be measured. The surface tension of these diluted systems is plotted against the dilution (concentration) and an extrapolation could be made to predict the approximate value of surface tension (surface energy) of the fluid food.
It is not a wild guess that most manufactured foods have a surface energy of approximately 30-40 mN/m because of the presence of protein, emulsifiers and other surface active ingredients. This explains why quartz glass (surface energy 54 mN/m) and stainless steel (surface energy 41 mN/m) are not the ideal materials to be used as a mould substrate. Polycarbonate has a surface energy of approximately 35 mN/m, probably in the same range of many food materials and is commonly used as a mould material in food manufacturing. Teflon has a very low surface energy of approximately 9 mN/m but tends to offer limited benefit in reducing surface stickiness compared to polycarbonate. Our recent research indicates that for a minimal surface adhesion and easy demoulding, a substrate with a surface energy of approximately 25 mN/m could be an ideal choice.
It should also be noted that, in addition to surface energy, some other causes may also influence the moulding and demoulding operation, specifically the mechanical interlocking, intermolecular liquid bridging and solid bridging. Surface irregularity and gurosity are the main causes of mechanical interlocking, where the contacting material penetrates and becomes mechanically locked in. Surface smoothing is the most effective way to minimise the mechanical interlocking (surface smoothing could also effectively reduce the effective surface area and therefore the total surface energy). Liquid bridging refers to the liquid filament formed between particles of contacting surfaces. Such liquid bridges are formed because of the surface tension of the liquid, which tends to wet contacting particles and hold them together. This occurs commonly during the moulding of semi-solid materials containing powdery components. The liquid component of the food (e.g. water, oil, viscous sugar solution) is capable of forming bridges and attaching food particles firmly to the moulding surface. Solid bridges are the result of molecule crystallisation (of such as sugar, fat) across the interface. Once solid bridges form, surface defects may become inevitable after demoulding.
Acknowledgement
Some materials of this article were obtained from the recent PhD work of Ms Esther Keijbets.