Microstructure characterisation of processed fruits and vegetables by complementary imaging techniques
- Like
- Digg
- Del
- Tumblr
- VKontakte
- Buffer
- Love This
- Odnoklassniki
- Meneame
- Blogger
- Amazon
- Yahoo Mail
- Gmail
- AOL
- Newsvine
- HackerNews
- Evernote
- MySpace
- Mail.ru
- Viadeo
- Line
- Comments
- Yummly
- SMS
- Viber
- Telegram
- Subscribe
- Skype
- Facebook Messenger
- Kakao
- LiveJournal
- Yammer
- Edgar
- Fintel
- Mix
- Instapaper
- Copy Link
Posted: 3 March 2011 | Adrian Voda, Jaap Nijsse & Gerard van Dalen, Unilever Research & Development and Henk Van As, Wagningen University and John van Duynhoven, Unilever Research & Development & Wagningen University | No comments yet
The assessment of the microstructural impact of processing on fruits and vegetables is a prerequisite for understanding the relation between processing and textural quality. By combining complementary imaging techniques, one can obtain a multi scale and real-time structural view on the impact of processing on fruits and vegetables.
Fruits and vegetables are considered rich sources of several essential dietary micro – nutrients, fibres and phytochemicals, therefore the intake of fruits and vegetables has been associated with a wide range of beneficial health effects. A main hurdle for consumers to raise their daily intake is the lack of convenience in preparing foods. The food industry has addressed this by offering the consumer dried fruits and vegetables that are re-hydrated shortly before consumption.
The assessment of the microstructural impact of processing on fruits and vegetables is a prerequisite for understanding the relation between processing and textural quality. By combining complementary imaging techniques, one can obtain a multi scale and real-time structural view on the impact of processing on fruits and vegetables. Fruits and vegetables are considered rich sources of several essential dietary micro - nutrients, fibres and phytochemicals, therefore the intake of fruits and vegetables has been associated with a wide range of beneficial health effects. A main hurdle for consumers to raise their daily intake is the lack of convenience in preparing foods. The food industry has addressed this by offering the consumer dried fruits and vegetables that are re-hydrated shortly before consumption.
The assessment of the microstructural impact of processing on fruits and vegetables is a prerequisite for understanding the relation between processing and textural quality. By combining complementary imaging techniques, one can obtain a multi scale and real-time structural view on the impact of processing on fruits and vegetables.
Fruits and vegetables are considered rich sources of several essential dietary micro – nutrients, fibres and phytochemicals, therefore the intake of fruits and vegetables has been associated with a wide range of beneficial health effects. A main hurdle for consumers to raise their daily intake is the lack of convenience in preparing foods. The food industry has addressed this by offering the consumer dried fruits and vegetables that are re-hydrated shortly before consumption. There is a big market for such products, which is currently dominated by dried soups. A major obstacle for further growth in this area is the relative poor quality of the rehydrated fruits and vegetables in the prepared food product. Most developments in drying of fruits and vegetables have been engineering-driven. In these approaches, emerging new drying technologies have been optimised to obtain rehydration properties and texture that match the original properties as much as possible. So far, no systematic and rational approach that considers the underlying microstructural features has been applied. A major barrier to embarking on such an approach has been the lack of adequate technologies that would enable decision making based on sound microstructural data.
Complementary imaging techniques
In order to investigate the microstructure of fruits and vegetables, complementary imaging techniques need to be employed. Depending on the resolution and moisture content, different techniques should be applied, as it is depicted in Figure 1. There, the anatomy of a fresh carrot is shown by Magnetic Resonance Imaging (MRI) while detailed microstructure of dried tissue is revealed by X-ray microtomography (μCT) and Scanning Electron Microscopy (SEM). Dried structures can be best assessed by μCT while high moisture states are well suited for MRI. One can probe the microstructure of samples noninvasively by means of μCT up to a few millimetres across with a resolution down to few micrometers and allows observations under environmental conditions without sample disturbing preparations1. The complete μCT pore structure of a dried carrot is presented in Figure 1. By using image analysis algorithms one can determine structural parameters like pore size distributions and pore connectivities. Going deeper in dissecting the porous matrix, SEM images reveal details at higher resolution of cellular architecture. Though SEM and μCT are outstanding for spatial resolution, NMR and MRI are remarkable for time resolution and for the versatility to assess various structure parameters under dynamic conditions. These imaging experiments can be carried out nondestructively and on optically non-transparent objects. Various possibilities of contrast can be exploited, revealing local differences in water density and mobility. In generic terms, MRI images of high moisture systems like fresh or re-hydrated fruits and vegetables can be recorded in less than a minute with a spatial resolution of tens of micrometer, depending on the application type. Hence, one can elegantly combine the high-spatial resolution of μCT imaging of dry structures with the local distribution of water in the re-hydrated state as seen by MRI. An example of such successive analysis on the same carrot piece is demonstrated in Figure 2. The dry carrot was first subject to μCT imaging (A) and the sample was shortly re-hydrated and imaged again by μCT (B) and MRI (C). Excellent agreement between images B and C was observed, showing the hydration status in terms of water density. Additional sub pixel data that carry water diffusion and mobility information is accessible by MRI. Together with dedicated image analysis tools, structural parameters from dry and re-hydrated states can be correlated and employed in predictive modelling.
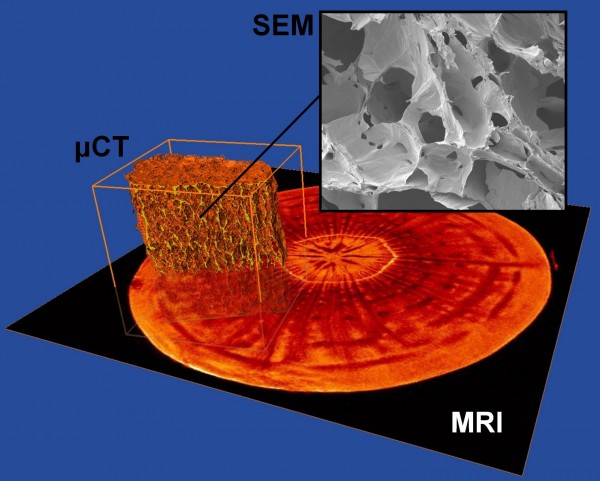
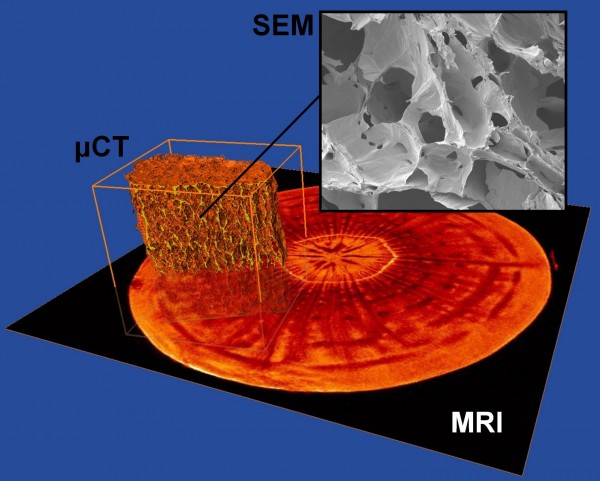
Figure 1 Multi-scale imaging approach employed
Since the microstructure upon drying determines the re-hydration behaviour, NMR spectroscopy methods can be used to assess the mobility of water in re-hydrated foods in a non-invasive manner. Compartment integrity and tissue permeability/tortuosity can be studied by NMR diffusometry. Molecular diffusion (self diffusion) of water molecules in re-hydrated plant material is restricted by surfaces with certain permeability that form pores with various sizes and shapes, connected by passages with different lengths and curvatures. The time-dependent behaviour of the self diffusion coefficient of water in such systems provides knowledge on the microstructure, like surface-to-volume ratio of the pore space, connectivity of the pore matrix and also the permeability of the pore walls2. Also, time-domain NMR relaxometry3 has been used to study horticultural systems and sophisticated approaches emerged from analysing water mobility and various populations of water residing in anatomically different sites. Relaxation parameters allow one to distinguish between free and immobilised water.
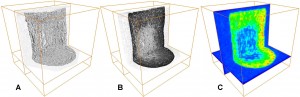
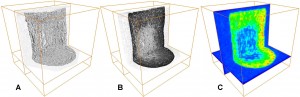
Figure 2 Successive imaging of the same piece of cortical tissue of freezedried
Assessment of dry microstructure
The microstructural impact of different drying methods affects the textural quality upon re-hydration. In one of our studies, we have prepared a series of carrot samples by freezing at different temperatures before freeze-drying. SEM and μCT images of fast (-196°C) and slowly (-28°C) frozen carrots are shown in Figure 3 A and B. In the SEM images, one can clearly distinguish different effects of freezing on cellular integrity. Freeze damage involves ice crystal growth which ruptures, pushes and compresses cells together. This effect is more pronounced in the slowly (-28°C) frozen tissue where the ice crystals grow bigger. Upon sublimation, a fingerprint of crystal size and shape is left. An overall sample view on differences in pore sizes between fast and slowly frozen carrots, as well as direction of ice crystal formation can be observed in Figure 3. The image of the fast frozen sample reflects the native cellular tissue. Morphology of re-hydrated structure Quality of re-hydrated fruits and vegetables is usually judged on sensorial properties. Texture, in particular, clusters together a series of sensations perceived by humans. Imaging and microscopy methods can reveal the underlying structural features that can help to establish structure-texture relations. Techniques like SEM and MRI together with NMR relaxometry and diffusometry are suitable to break into details of re-hydrated structures. At sub-μm level, SEM images (not shown here) reveal the hydration/swelling state of cells and cell walls, which may not be accessible by MRI resolution. The signal intensity in the MRI images shown in Figure 3 C is directly proportional with water content. Differences between initially dry microstructures are visible on re-hydrated state. The carrot frozen at -196°C shows a native structure pattern after re-hydration. The time needed to obtain such images is of the order of minutes while achieving a resolution of tens of μm. With no requirements for sample preparation, MRI offers a fast way to obtain water distributions in two- and three-dimensional space.
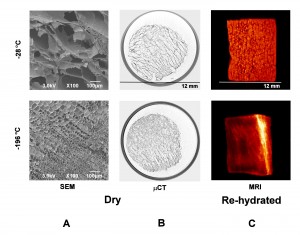
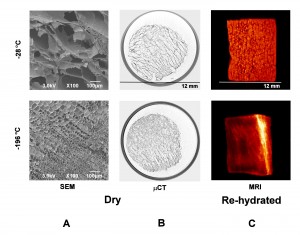
Figure 3 SEM, μCT and MRI images of cortical
Additional information about the re-hydrated microstructure of carrots can be obtained from NMR relaxation and diffusion measurements. Thermal processing like blanching and freezing result in a significant increase in membrane permeability and in some cases cells break and form a connected network, which makes the re-hydrated system behave more like a sponge characterised by a certain tortuosity. These factors affect the mobility of water molecules and the exchange process between water protons and tissue macromolecules, which can be detected by NMR relaxation experiments. The knowledge on the compartments in re-hydrated carrots can be complemented by analysing the self-diffusion behaviour of water over a variable time scale, which is translatable into distances travelled by water molecules within the porous media. Samples frozen at lower temperature show more anisotropy, as was observed by measuring different diffusion constants along longitudinal and transverse directions, suggesting less structural damage (data not shown). The tortuosity parameter obtained from NMR diffusion data is shown in Figure 4 together with texture measurements. Slowly frozen carrots show almost no tortuosity, which means that water molecules can easily travel through a well connected porous structure. No significant difference was observed between slowly frozen blanched and non-treated carrots in terms of texture and tortuosity, clearly demonstrating the detrimental effect of ice crystal growth.
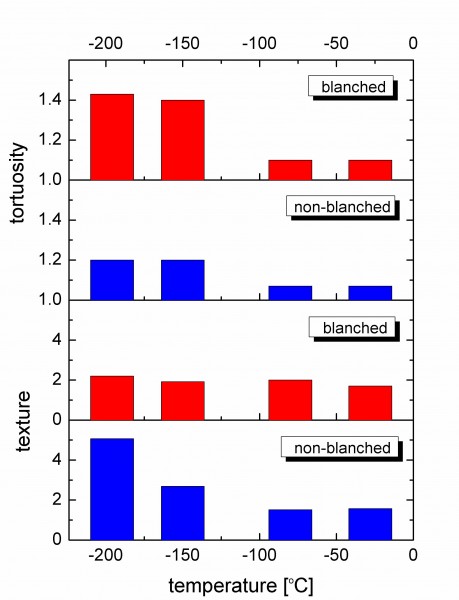
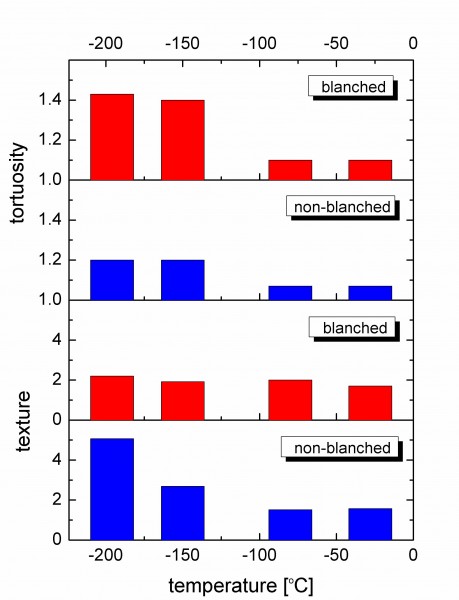
Figure 4 Texture and tortuosity in re-hydrated carrots frozen at different
Macroscopic transport of water during re-hydration
Re-hydration of dry fruits and vegetables immersed in water can be monitored in real-time and in-situ by means of fast MRI techniques. An example is shown in Figure 5, where two-dimensional images (one millimetre thickness and in-plane resolution of 156 μm) were recorded with a time resolution of eight seconds. Such moisture ingress profiles are now being used by us to verify predictive microstructure-based models for water transport in processed fruits and vegetables.
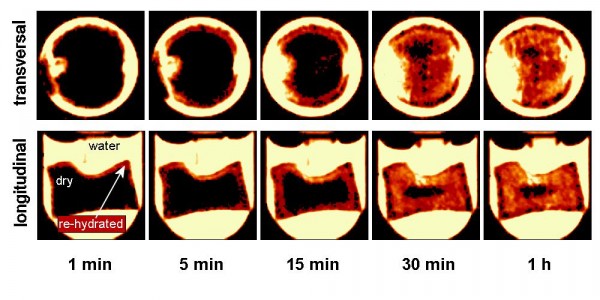
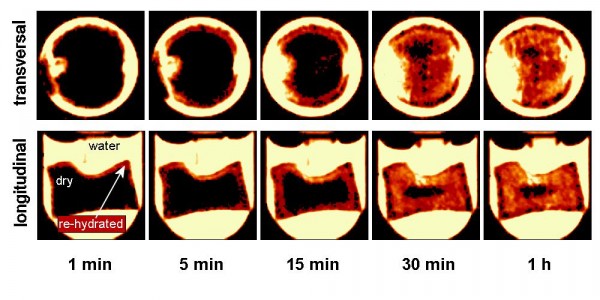
Figure 5Water ingress in a dried carrot piece as a function of hydration
Conclusions
Combination of complementary imaging techniques yields a multi-length scale view on fruits and vegetables microstructures. The complexity of phenomena which occur during processing can be resolved by complementary information emerging from these techniques. Water ingress during re-hydration can be quantitatively tracked by MRI in-real time and in-situ.
Acknowledgement
Parts of this work were supported by the Dutch Food and Nutrition Delta programme (project FND08001).
References
1. 3-D imaging, analysis and modelling of porous cereal products using X-ray microtomography, van Dalen, G., Notenboom, P., van Vliet, L. J., Voortman, L., & Esveld, E., Image Analysis Stereology, 2007, 26, 169–177
2. Intact plant MRI for the study of cell water relations, membrane permeability, cell-to-cell and long distance water transport, Van As, H., Journal of Experimental Botany, 2007, 58, 4, 734-756
3. Time-Domain NMR Applied to Food Products, van Duynhoven, J., Voda, A., Witek, M., & Van As. Ann. Reports on NMR Spectroscopy, 2010, 145-197
About the Author
Dr. Adrian Voda is a research scientist in the Advanced Measurements and Data Modelling group at Unilever R&D in the Netherlands. He is a physicist with a PhD degree obtained at the RWTH Aachen University in Germany and he joined Unilever in 2008. His expertise is in the area of NMR spectroscopy and imaging applied in material science. He (co)-authored scientific publications on NMR applications in polymer science, chemical engineering and food science.