Enzymatic detoxification of mycotoxins for healthy food
- Like
- Digg
- Del
- Tumblr
- VKontakte
- Buffer
- Love This
- Odnoklassniki
- Meneame
- Blogger
- Amazon
- Yahoo Mail
- Gmail
- AOL
- Newsvine
- HackerNews
- Evernote
- MySpace
- Mail.ru
- Viadeo
- Line
- Comments
- Yummly
- SMS
- Viber
- Telegram
- Subscribe
- Skype
- Facebook Messenger
- Kakao
- LiveJournal
- Yammer
- Edgar
- Fintel
- Mix
- Instapaper
- Copy Link
Posted: 23 June 2014 | Petr Karlovsky, Head of the Molecular Phytopathology and Mycotoxin Research Unit, University of Göttingen | No comments yet
Mycotoxins are poisonous fungal metabolites that occur in food commodities colonised with filamentous fungi and in food products contaminated during processing and storage. Intake of mycotoxins with food poses a health risk to the consumer and legal limits for maximum levels of major mycotoxins in food have therefore been established worldwide. Compliance with these limits poses a challenge to food industry because good integrated plant protection, adequate storage and good manufacturing practices are often insufficient to keep mycotoxin levels below the limits…
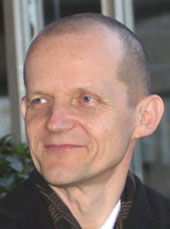
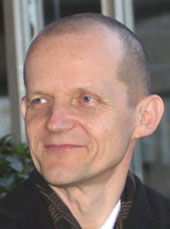
Petr Karlovsky, Head of the Molecular Phytopathology and Mycotoxin Research Unit, University of Göttingen
New options for mycotoxin management with the help of biotechnology rely on enzymatic activities that detoxify mycotoxins enzymatically. Crop plants can be engineered to detoxify mycotoxins in the field. Purified detoxification enzymes or genetically engineered microorganisms (GMOs) producing such enzymes can detoxify mycotoxins during storage and processing of raw materials in food production. GMOs do not have to be declared on food products in the EU when the microorganisms were removed from the product or the enzymes synthesised by GMOs were used merely as processing aid. The situation is similar to numerous consumer goods on the market produced with the help of purified vitamins and enzymes produced by GMOs. Enzymatic detoxification of mycotoxins offers an opportunity to make food healthier with the help of genetic technology without jeopardising market share in spite of the adverse attitude of European consumers toward GMOs.
Chemical composition of agricultural commodities used in food production seldom matches the demands on healthy and balanced nutrition. The vast majority of undesirable compounds in foods originate from biosynthetic pathways of crop plants and microorganisms associated with living plants and stored plant products. Food pollutants consisting of manmade chemicals introduced into the food chain deliberately (e.g. pesticide) or unintentionally (e.g. contaminants released by packaging materials) or generated by chemical reactions during food processing (e.g. acrylamide) received prominent media attention but the potential health damage they may cause is not nearly as relevant as the health risk caused by contaminants of natural origin. Natural products that occur as contaminants of food include metabolites of plants, bacteria and fungi. Fungal toxic metabolites, called mycotoxins, have been reported to contaminate food since the Middle Ages. While acute and even lethal poisoning by mycotoxins occasionally occurs, major concerns regarding mycotoxin exposure today are long-term effects of chronic exposure such as distortion of hormone balance, suppression of the immune system, and the ability of certain mycotoxin to cause cancer.
In order to reduce the exposure of consumers to mycotoxins, legislatures limiting the amount of mycotoxins in raw materials and food products have been established worldwide. In Europe, assessments of risks posed by individual mycotoxins to human health are generated by the European Food Safety Authority (EFSA). The results of the assessments, published as opinions, are the basis of legislative control of mycotoxin levels in the European Union.
Legal limits for mycotoxin levels in food protect the consumer as long as they are observed. Full control of mycotoxin levels along the food chain is not feasible. In contrast to the control of synthetic contaminants, which can be achieved by enforcing good manufacturing practices and hygiene standards, only indirect means of the control of mycotoxin accumulation in food commodities are available. The efficiency of these measures is limited. Mycotoxin prevention during plant production has focused on the elimination of fungal inoculum, suppression of plant infection and inhibition of fungal growth by agronomic practices such as tillage and seed coating, growing resistant cultivars and using fungicides in the field. Optimised storage conditions and chemical preservatives protect stored commodities from spoilage. All these measures are effective to some extent, particularly when used in combination. Under strong infection pressure, however, growers fail to meet legal requirements for mycotoxin levels. Grain that exceeds maximal legal levels is unfit for food and feed manufacturing. Even when mycotoxin levels meet legal limits, further reduction is desirable from the public health perspective, particularly regarding mycotoxins that are suspected or proven carcinogens.
A promising strategy for the reduction of mycotoxin content in food products is biological detoxification, which is defined as enzymatic degradation of mycotoxins or modification of their structure that leads to less toxic products1. Organisms that possess detoxification activities can rarely be used for detoxification of mycotoxins in food commodities directly but they serve as a source of genes encoding suitable enzymes that can be expressed in other microorganisms or in crop plants. Enzymes prepared from genetically modified organisms and used in food processing do not have to be declared as ‘GM component’ on the final product in the European Union. Such enzymes are used on a large scale in food products sold on European markets without raising concerns about GM organisms, for example in bakery products and cheeses.
The following will describe enzymatic activities suitable for the detoxification of major mycotoxins in food, depict related industrial efforts and outline possible future developments.
Detoxification of trichothecenes
Trichothecenes are mycotoxins produced by a number of phytopathogenic Fusarium species, by Trichoderma species used in the biological control of plant diseases and by further fungal genera not relevant for food production. Major trichothecenes in cereals and maize are deoxynivalenol, nivalenol and their acetylated derivatives 3-acetyl-deoxynivalenol, 15-acetyl-deoxynivalenol and 4-acetyl-nivalenol, known as fusarenon X. The search for trichothecene-detoxifying microorganisms, which began more than 30 years ago and involved numerous academic laboratories and defence-related research projects, was marked by failures and setbacks1. In the last 15 years, a series of remarkable discoveries invigorated the field2. Detoxification activities for trichothecenes described so far are summarised in Figure 1, which shows deoxynivalenol as major trichothecene in cereal grains in temperate climate.
Complete mineralisation of trichothecenes by a pure bacterial culture was described already in 1983 but the activity was lost3. Very recently, the laboratory of Seiya Tsushima in the National Institute for Agro-Environmental Sciences in Ibaraki, Japan, isolated new several bacterial strains that appear to completely demineralise deoxynivalenol4. Full details of the discovery are yet to be disclosed.
The epoxide group of trichothecenes is their key toxicity determinant. Detoxification of trichothecenes by intestinal and ruminal microflora due to reductive deepoxidation was established in the 1980s and has been studied extensively since2. It took 15 years until the first pure bacterial strain capable of reductive deepoxidation of trichothecenes under anaerobic conditions was isolated5. The enzyme(s) and gene(s) involved in the process remain unknown. The intact bacterium was used as a component of a feed additive but its application in food industry appears unlikely.
Search for detoxification of trichothecenes intensified in the last decade. Various environments were used as sources of microflora for enrichment cultures, such as chicken intestine, fish digesta and most recently even human faecal microflora6. Many studies were successful in achieving biotransformation of deoxynivalenol by mixed bacterial cultures. The most recent and surprising result was the isolation of pure bacterial cultures, assigned to six Gram-positive and Gram-negative genera, that were capable of detoxifying deoxynivalenol by reductive deepoxidation under aerobic conditions7. These strains are promising as a source of genes and enzymes for the detoxification of trichothecenes in food production.
A second toxicity determinant of trichothecenes is the hydroxyl group on C3. All modifications of C3-OH of deoxynivalenol studied so far lead to the reduction of toxicity. The modifications studied most extensively were acetylation, catalysed by the product of Tri101 gene of Fusarium graminearum and related Fusarium species, and glycosylation, catalysed by plant UDP-glycosyltransferases. Acetylation of trichothecenes on C3-OH by trichothecene-producing fungi is believed to protect the fungi from their own products8. Glycosylation of trichothecenes is a component of the defence response of plants infected with pathogenic Fusarium species9.
Epimerisation of hydroxyl on C3-OH (Figure 2), which we hypothesise to proceed via an oxidised intermediate which is stereospecifically reducted2, leads to 3-epi-deoxynivalenol, which is nontoxic (Gareis and Karlovsky, unpublished). The chemistry of the process is not understood and the enzymatic activities involved remain unknown.
Potential for trichothecene detoxification in food production
Expression of Tri101 in a genetically engineered wheat variety aiming at the reduction of deoxynivalenol content in flour was attempted by seed industry but the development was abandoned2. The use of an enzyme with sub-optimal activity, encoded by a Tri101-homologue isolated from a Fusarium species that does not produce deoxynivalenol, may have been partly responsible for the failure, apart from animosity against genetically engineered crops that is commonplace in Europe2. As far as the author is aware, the strategy is not actively pursues by the industry in the moment.
Acetylation of trichothecenes in yeast expressing Tri101 is feasible8. Baker’s yeast and brewer’s yeast are example of organisms that can be enhanced by expressing Tri101 to detoxify deoxynivalenol, T-2 toxin and other toxic trichothecenes in dough, beer and other products. Labelling food products processed using enzymes extracted from genetically modified organisms or even living microbial strains containing genes from other organisms is not mandatory. For example, yeast used in beer brewing is regarded as ‘processing aids’. If the yeast is removed from the product, as is the case with pale lager, pale draught beer and light beer, labelling of the beer as a product of genetically modified organisms is not be required by law. Even strict Bavarian Purity Law (in German ‘Reihnheitsgebot’), which is voluntarily observed by most beer manufacturers in Germany, would not be violated by the use of a genetically modified yeast strain. Major problems of the strategy are firstly, the fact that trichothecenes acetylated at C3-OH do not completely lose toxicity10 and secondly, the possibility of hydrolysis of the acetyl group during passage through gastrointestinal tract11,12.
Glycosylation of trichothecenes on C3-OH likely prevents the uptake of these toxins by living cells; it is therefore regarded as detoxification12. A feeding study with rats showed that bioavailability of deoxynivalenol-3-O-glucoside is limited; the authors concluded that the derivative possesses considerably lower toxicological relevance than non-glycosylated deoxynivalenol13. Because glycosylation of trichothecenes is a component of defence response of crop plants to fungal infection, deoxynivalenol-3-O-glusocide occurs in cereal products9. Availability of yeast strains expressing trichothecene-3-O-glysosylase of plant origin14 and yeast species which glycosylate trichothecenes naturally15 opened the opportunity to use glycosylation of trichothecenes as a detoxification reaction in food processing.
Both acetylation and glycosylation of C3-OH are potentially reversible. Irreversible detoxification reactions are known but suitable genes and enzymes are not available. Last year’s re-discovery of bacterial activities that led to complete mineralisation of trichothecenes4, which had been reported 30 years earlier3 but irrevocably lost1, as well as the recent discovery of reductive deepoxidation under aerobic conditions7, has raised hopes that these activities will be available for food production in the future.
Enzymatic detoxification of mycotoxins: chances and hurdles for commercialisation
The feasibility of enzymatic detoxification of trichothecenes, which belong to most important mycotoxins in countries of the Northern temperate zone, has been established in vitro and demonstrated in genetically modified crops. Detoxification of further mycotoxins has been studied since the 1980s. Identification of enzymes detoxifying aflatoxins and zearalenone are significant achievements with potential impact on food production. The major obstacle for the implementation of the technology in crop production has been public opposition against genetic technology, nourished by ideologically and economically motivated lobbies in Europe and elsewhere. As a result, many food companies restricted the use of GMOs to manufacturing vitamins, enzymes and other processing aids, the origin of which does not have to be declared on food products. Tropical and subtropical countries which suffer from widespread mycotoxin contamination of food and whose markets are not indoctrinated by anti-GMO propaganda are likely to be the first to benefit from enzymatic detoxification of mycotoxins in crop production and food manufacturing. In the long term, however, even Europe cannot afford to ignore the potential of biotechnology in counteracting mycotoxins and other poisons of natural origin in food.
References
- Karlovsky P (1999) Biological detoxification of fungal toxins and its use in plant breeding, feed and food production. Nat Toxins 7: 1–23
- Karlovsky P (2011) Biological detoxification of the mycotoxin deoxynivalenol and its use in genetically engineered crops and feed additives. Appl Microbiol Biotechnol 91: 491–504
- Ueno Y, Nakayama K, Ishii K, Tashiro F, Minoda Y, et al. (1983) Metabolism of T-2 toxin in Curtobacterium strain 114-2. Appl Environ Microbiol 46: 120–127
- Ito M, Sato I, Ishizaka M, Yoshida S, Koitabashi M, et al. (2013) Bacterial cytochrome P450 system catabolizing the Fusarium toxin deoxynivalenol. Appl Environ Microbiol 79: 1619–1628
- Fuchs E, Binder EM, Heidler D, Krska R (2000) Characterisation of metabolites after the microbial degradation of A- and B-trichothecenes by BBSH 797. Mycotoxin Research 16: 66–69
- Gratz SW, Duncan G, Richardson AJ (2013) The human fecal microbiota metabolizes deoxynivalenol and deoxynivalenol-3-glucoside and may be responsible for urinary deepoxy-deoxynivalenol. Appl Environ Microbiol 79: 1821–1825
- Islam R, Zhou T, Young JC, Goodwin PH, Pauls KP (2012) Aerobic and anaerobic de-epoxydation of mycotoxin deoxynivalenol by bacteria originating from agricultural soil. World J Microbiol Biotechnol 28: 7–13
- Kimura M, Kaneko I, Komiyama M, Takatsuki A, Koshino H, et al. (1998) Trichothecene 3-O-acetyltransferase protects both the producing organism and transformed yeast from related mycotoxins. Cloning and characterization of Tri101. J Biol Chem 273: 1654–1661
- Schweiger W, Boddu J, Shin S, Poppenberger B, Berthiller F, et al. (2010) Validation of a candidate deoxynivalenol-inactivating UDP-glucosyltransferase from barley by heterologous expression in yeast. Mol Plant Microbe Interact 23: 977–986
- Pinton P, Tsybulskyy D, Lucioli J, Laffitte J, Callu P, et al. (2012) Toxicity of deoxynivalenol and its acetylated derivatives on the intestine: differential effects on morphology, barrier function, tight junction proteins, and mitogen-activated protein kinases. Toxicol Sci 130: 180–190
- Berthiller F, Krska R, Domig KJ, Kneifel W, Juge N, et al. (2011) Hydrolytic fate of deoxynivalenol-3-glucoside during digestion. Toxicol Lett 206: 264–267
- Maresca M (2013) From the gut to the brain: journey and pathophysiological effects of the food-associated trichothecene mycotoxin deoxynivalenol. Toxins 5: 784–820
- Nagl V, Schwartz H, Krska R, Moll W-D, Knasmueller S, et al. (2012) Metabolism of the masked mycotoxin deoxynivalenol-3-glucoside in rats. Toxicol Lett 213: 367–373
- Schweiger W, Pasquet J-C, Nussbaumer T, Paris MPK, Wiesenberger G, et al. (2013) Functional characterization of two clusters of Brachypodium distachyon UDP-glycosyltransferases encoding putative deoxynivalenol detoxification genes. Mol Plant Microbe Interact 26: 781–792
- McCormick SP, Price NPJ, Kurtzman CP (2012) Glucosylation and other biotransformations of T-2 toxin by yeasts of the Trichomonascus Appl Environ Microbiol 78: 8694–8702
About the author
Prof. Dr. Petr Karlovsky is Head of the Molecular Phytopathology and Mycotoxin Research Unit at the University of Göttingen, Germany. He studied biochemistry in Brno, Czech Republic, and obtained PhD degree in Institute of Biophysics in the same city. After his studies he moved to Germany to work at universities in Hohenheim and Göttingen with an interruption for a visiting professorship at the University of Connecticut, USA. Before accepting a faculty position in Goettingen, P. Karlovsky worked as a Research Manager with DuPont/Pioneer Hi-Bred in Johnston and Newark, USA. His laboratory investigates biological functions of secondary metabolites; recent projects focus on the detoxification and ecological functions of mycotoxins. Prof. Karlovsky is Vice President of the Society for Mycotoxin Research, was nominated for two ILSI Expert Groups, is a member of the Mycotoxin Research and Journal of Phytopathology editorial boards and serves as a reviewer for granting agencies of Austria, Czech Republic, Germany, Israel, Portugal, Singapore and USA. He has co-authored numerous scientific publications and is listed as a co-inventor on several patents.