Getting a grip on water holding capacity
- Like
- Digg
- Del
- Tumblr
- VKontakte
- Buffer
- Love This
- Odnoklassniki
- Meneame
- Blogger
- Amazon
- Yahoo Mail
- Gmail
- AOL
- Newsvine
- HackerNews
- Evernote
- MySpace
- Mail.ru
- Viadeo
- Line
- Comments
- Yummly
- SMS
- Viber
- Telegram
- Subscribe
- Skype
- Facebook Messenger
- Kakao
- LiveJournal
- Yammer
- Edgar
- Fintel
- Mix
- Instapaper
- Copy Link
Posted: 9 March 2015 | Ruud van der Sman, Food & Biobased Research | No comments yet
In the design of food products which are high in protein or fibre, it is important for their various properties to control how much water is held by the food material. This water holding capacity (WHC) relates to many sensory, health, nutritional and convenience properties.
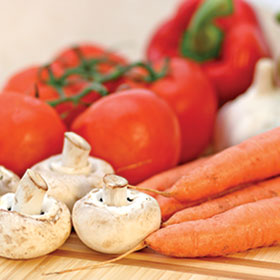
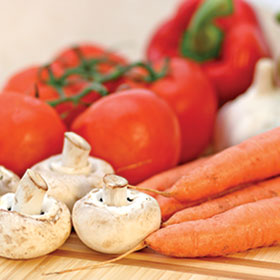
For example, WHC is a measure of the juiciness of foods like meat. Furthermore, recent research indicates that WHC is an important property for digestion and satiety of foods that are rich in fibre. Thermal processing of protein-rich food is accompanied by a loss of water (containing various nutritional components), so better control of WHC means reducing loss of the nutritional value. Additionally, WHC controls the rehydration rate of dried vegetables, as in instant soups.
Despite its importance for many properties of food, WHC is still poorly understood in food science. Above all, scientists have not agreed on a good definition and a well established experimental method to measure it. Wageningen UR Food & Biobased Research has developed an expertimental method to measure WHC. By using the theory of polymer physics describing the water retention of gels made from synthetic polymers (as applied, for example, in nappies), we have circumvented the ill-posedness of the WHC concept. Our experimental method to measure WHC is also centred around this theory.
Flory-Rehner theory for water retention in gels
Polymer physics states that for a material to retain solvent, the polymer should form a network, i.e. it has to be a gel. This equally holds for foods. Food materials, which are said to have WHC properties, are indeed gels made from protein or soluble fibres, or natural foods which still have tissue structure like meat, vegetables or mushrooms. In these natural foods the gel network is formed by either (muscle) proteins or by the cell wall material – a matrix of several crosslinked fibers. In polymer physics, the retention of solvent in a polymer network is traditionally described by the Flory-Rehner theory. Food & Biobased Research has applied this theory to explain the WHC of various food materials.
The Flory-Rehner theory states that the water retention of a gel is determined by a gel pressure or swelling pressure, which is the sum of several independent contributors. These are: (1) the thermodynamic interaction between solvent and polymer, (2) the elasticity of the polymer network, and (3) the electrostatic repulsion generated by charged polymers. These contributions are sketched schematically in Figure 1. According to the theory, the swelling pressure can be written as a sum of these three contributions:
Pswell = Pmix + Pelas + Pion
Under a mechanical load, as expressed by an external pressure, the swelling pressure equals the external pressure.
The first contribution to the swelling pressure (Pmix) is described by the well-known Flory-Huggins theory. We have shown recently that this describes the water sorption of a wide variety of food materials. The second contribution (Pelas) is due to the stretching of the polymer network by mechanical loads, and can be described by various models from the hyperelastic models for elastomers, such as the Neo-Hookean model or the Rehner model.
Various food polymers like proteins and several food fibres have side groups, which can be charged under certain pH conditions. The charged polymers (polyelectrolytes) will repel each other, allowing more solvent to be retained by the gel network. The electrostatic repulsion of these polyelectrolytes is modulated by pH, counter-ions and added salts. In polymer physics, several models are used for the description of the thermodynamic state of polyelectrolytes, i.e. Pion. At low salt concentrations, the ionic contribution is dominated by the charged polyelectrolytes, which is described by the Donnan theory. At salt concentrations as are normal in processed foods, the ionic contribution is dominated by the salts, via the Raoult or Pitzer theory1.
Knowledge of the individual contributions to the swelling pressure teaches us how various factors influence the WHC. This knowledge can again be used to design the WHC of various gel-like foods. The mixing pressure is determined by how hydrophilic the food material is, which is expressed by the Flory-Huggins interaction parameter.
During heating, food proteins can unfold and expose their hydrophobic parts to water, consequently developing a dislike of water. This causes an increase of the swelling pressure and the proteins squeeze the water out of the matrix. During the cooking and frying of meat, this leads to the generation of juice. The WHC of cooked meats can be retained by careful control of temperature and time during cooking, as is currently performed by the top chefs in Michelin restaurants applying the sous-vide cooking technique3. The Flory-Huggins theory also teaches us that the water retention can be increased by the addition of water loving, low molecular weight solutes like carbohydrates, polyols and amino-acids.
The elastic contribution to the swelling pressure is controlled by a number of interconnections (also known as crosslinks) in the gel network. One can control the number of crosslinks in food gels via special enzymes, or via the temperature and cooling rate during gelation.
The Donnan-theory describing the ionic contribution to the swelling pressure teaches us how added salts and pH influence the WHC. Their influence is evident for meat products, whose WHC can be enhanced via brining with salts and acids. Depending on the pH and amount of ions (salts) in the brine, the proteins acquire an electric charge, which makes the proteins repel each other and makes room for more water to be retained. Several food fibres can also be charged via change of pH or amount of ions.
Experimental method
Our newly developed experimental procedure is directed at determining the various parameters present as described in the Flory-Rehner theory2. To this end we subject the food gel to a wide range of mechanical loads and correlate the water retention with our theory. Our means of applying an external pressure is via centrifugation. During centrifugation water is allowed to escape from the centrifuge tube via a membrane filter. By careful measurement of the mass of the food gel before and after centrifugation, we determine the WHC for various centrifugation speeds. Often, before the centrifugation experiment begins, the food gel is subject to a pre-treatment – which can be heating, change of pH or ionic strength via brining, or impregnation with solutes.
The osmotic contribution can be obtained independently via moisture sorption measurements. The measured sorption isotherm can be interpreted via the Flory-Huggins theory, which obtains an accurate estimation of the Flory-Huggins interaction parameter, stating the hydrophilicity of the food material. For many food components the interaction parameter is already known. Therefore, for many foods we can predict the osmotic pressure (Pmix) via the food composition. Because we can obtain the osmotic pressure via independent measurements, we can derive the elastic contribution from our centrifugation experiments via simple subtraction of the osmotic pressure from the total swelling pressure. This allows us to obtain an accurate determination of the number of crosslinks in the gel network.
Analysis of the WHC of carrot and mushroom
In this article we will illustrate the use of the theory and experimental method by showing how we have determined the WHC of carrots and mushrooms to optimise their rehydration rate in instant soups.
First, we have determined the water content of the food materials as a function of the swelling pressure by means of the centrifugation experiments. To connect the measurements to theory, we have converted the water content into the volume fraction of polymers present in the gel fp. The results of the centrifugation experiments are shown in Figure 2.
Like other natural food products, the investigated carrots and mushrooms have a neutral pH and low ionic strength. Consequently, the ionic contribution to their swelling pressure is negligible. The osmotic pressure is determined via independent measurement of the sorption isotherms, which are depicted in Figure 3. In this figure we have compared the measurements with our predictions of the Flory-Huggins theory, which shows that the predictions are in good agreement with the measurements.
After subtracting the osmotic pressure from the swelling pressure, we obtain the elastic pressure as a function of the polymer volume fraction, as shown in Figure 4. We have fitted this curve with the Flory-Rehner theory, rendering accurate estimates of the elastic material properties.
Recently, we have discovered that several foods like meat and freeze-dried vegetables have a distinct pore space, which must be viewed differently from the gel phase, making up the meat fibres or cell wall material4. In the pore space, the water is held by capillary forces and its (capillary) pressure is described by the Laplace law. We are currently working on an extension of the theory to include this pore water for the WHC of these foods.
Use of the theory in food design
When designing gel-like foods the Flory-Rehner theory is a powerful tool to tailor their sensory and health properties. One of these sensory traits is the juiciness of foods. In the mouth we exert some mechanical pressure on the food, which will expel some of its water – which we sense orally as juiciness.
Via the Flory-Rehner theory we know how WHC can be controlled by processing conditions like temperature, pH, and ionic strength. Furthermore, via careful process control or addition of enzymes one can control the number of crosslinks during the gelation of the food material, which has a profound influence on the WHC and juiciness. Finally, the juiciness can be influenced via the food formulation, such as fibre enrichment and addition or replacement of solutes. Often sugar or salt are present in the food formulation. With help of the theory one can efficiently search for other solutes, which have less impact on consumer health.
Juiciness is particularly important for novel protein foods made from plant resources. It appears that in their native state, plant proteins are more hydrophobic than proteins from animal sources. Hence, from the theory we understand now that the big challenge for foods based on plant proteins is to achieve the juiciness of meat products.
WHC is also important for other textural sensations like crumbliness and spreadability. The amount of water absorbed by the food contributes strongly to the dissipation of mechanical energy excerted during biting and mastication.
The digestive properties of foods rich in proteins or fibres are also better understood with our theory. In the mouth, the food will hydrated by saliva. The WHC of the bolus is one of the determinants for the moment of swallowing. In the stomach the enzymes and the low pH will impart significantly the WHC of the food, via attack of the crosslinks and change of the charge of biopolymers like proteins and various fibres like pectin. The swelling of food in the stomach is one of the signals for the satiety sensation. Novel foods meant to trigger satiation are designed to gel in the stomach. Here, food formulators use the effect of stomach pH on chargeable fibres like pectin or alginate. At neutral pH, these fibres are soluble, but form strong gels at gastric conditions with low pH.
In the small and large intestine the swelling of the food material promotes peristaltic movements, which improves residence times. This again is said to be beneficial for nutrient absorption and helping prevent constipation and intestinal disorders.
To Summarise, we have acquired a good theorectical understanding of water retention by food gels and experimental procedures for measuring it. These are valuable tools for designing foods with respect to several sensory and digestive properties, or their reformulation with more healthy ingredients, while retaining desired sensory and textural properties.
References
- Van der Sman, RGM (2012). Thermodynamics of meat proteins. Food Hydrocolloids 27(2), 529-535.
- Van der Sman, RGM, Voda, A, Khalloufi, S and Paudel, E (2013). Hydration properties of vegetable foods explained by Flory-Rehner theory. Food Research International 54(1), 804-811.
- Van der Sman, RGM (2013). Modelling cooking of chicken meat in industrial tunnel ovens with the Flory-Rehner theory. Meat Science 95(4), 940-57.
- Van der Sman, RGM et al. (2014). Multiphysics pore-scale model for the rehydration of porous foods. Innovative Food Science & Emerging Technologies 24, 69-79.
About the author
Ruud van der Sman has a MSc in Applied Physics from Delft University of Technology, and a PhD in Agricultural Engineering from Wageningen University. He works as a Senior Researcher at Food & Biobased Research, and as a part-time Assistant Professor at Food Process Engineering – both part of Wageningen UR (University & Research centre). His research interests comprise of soft matter physics of food materials, computer modelling of food structures at the micrometre scale, and physiology modelling. His expertise in meat comprises of the thermodynamics of water holding capacity, heat and mass transfer, and post-mortem physiology.