High Pressure Processing: Opportunities to produce healthy food products and ingredients
- Like
- Digg
- Del
- Tumblr
- VKontakte
- Buffer
- Love This
- Odnoklassniki
- Meneame
- Blogger
- Amazon
- Yahoo Mail
- Gmail
- AOL
- Newsvine
- HackerNews
- Evernote
- MySpace
- Mail.ru
- Viadeo
- Line
- Comments
- Yummly
- SMS
- Viber
- Telegram
- Subscribe
- Skype
- Facebook Messenger
- Kakao
- LiveJournal
- Yammer
- Edgar
- Fintel
- Mix
- Instapaper
- Copy Link
Posted: 27 October 2014 | Vibeke Orlien, Head of Food Chemistry, Research Station, University of Copenhagen / Francisco J. Barba, Assistant Professor – Food Science and Nutrition, University of Valencia / Roman Buckow, Stream Leader, CSIRO / Netsanet Shiferaw Terefe, Research Scientist, CSIRO Animal, Food and Health Sciences Division | 2 comments
High pressure processing (HPP) has emerged as a new, non-thermal, and additive-free preservation technology with a wide range of applications in the food industry. The next stage is to identify the opportunities HPP presents for the production of healthy and safe food products and ingredients. This article is a short survey describing the recent findings in the applications of HPP to extract bioactive components from plant food materials, to reduce the allergenicity of food products and to obtain healthier products with reduced salt and fat and improved fatty acid composition. The studies so far indicate that HPP has significant potential for such applications and, thus, there is considerable opportunity for the food industry to exploit these opportunities to bring the next generation of HPP food products to the market.
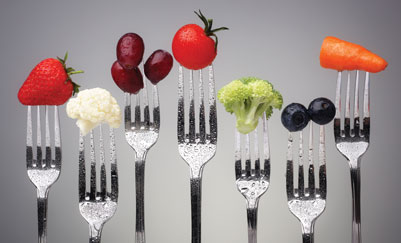
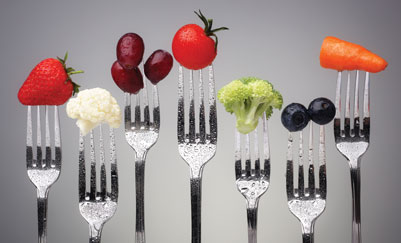
It has been scientifically and commercially proven that high pressure processing can produce microbiologically safe and stable products with improved quality characteristics such as enhanced flavour and colour retention1. Apart from food preservation applications, more recent studies have focused on the effects of high pressure processing on health attributes and allergenic potential of foodstuff to develop the next generation of convenience foods. HPP has shown encouraging potential to manipulate the extractability, allergenicity, and functionality of micronutrients and components in a diverse variety of foods2-4.
Typically, high pressure processing involves subjecting food to hydrostatic pressures of 300 to 700 MPa for periods of a few minutes. The efficacy of high pressure processing is governed by Le Chatelier’s principle stating that chemical reactions or physical processes associated with a decrease in molecular volume are favoured, whilst those accompanied with a volume increase are inhibited. This means that covalent bonds are not broken by high pressure processing and therefore small, low molecular weight molecules in food, such as aroma compounds and vitamins, are rarely affected. On the other hand, macromolecules, such as proteins and starches, can change their native structure during high pressure processing, opening possibilities for structural modifications that may lead to novel functionalities.
High pressure processing is characterised by three processing parameters: pressure (P), temperature (T), and exposure time (t). These three processing parameters allow great versatility in the design of the process and the resulting impact on the food material. The superiority of high pressure processing over heat processes (where heat is often transferred through slow conduction processes) is that pressure is transmitted instantaneously and uniformly throughout the system, independent of the size and geometry of the food product. This can reduce processing time, processing energy, and the risk of over processing of food products.
Extraction of bioactive compounds
The interest in secondary metabolites recovery from plant food materials and by-products is increasing due to their health and nutraceutical benefits. Currently, many studies have focused on agricultural and industrial wastes in the search for natural antioxidants. According to the Food and Agriculture Organization (FAO), approximately one third of the edible parts of food produced for human consumption is lost or wasted globally5. This amounts to about 1.3 billion tonnes per year and accounts for both food processing wastes and by-products and food waste at retail or consumer ends. Plant material waste and by-products are excellent sources of secondary metabolites such as carotenoids and phenolic compounds and can be used to obtain functional extracts rich in bioactive compounds for use in several applications (e.g. nutraceuticals, antimicrobials, colourants).
There are various studies focusing on HPP-assisted extraction of phenolic compounds from different plant food materials and by-products. For example, Jun et al.6 compared the effects of HPP (100-600 MPa / 20°C / 1-10 min) and conventional extraction methods (heat reflux: 85ºC / 45 min; extraction at room temperature; ultrasonic extraction: 50Hz (frequency) / 250W (power) / 20-40ºC / 90 minutes) on the extraction of tea leaves using different solvents (acetone, methanol, ethanol and water). They obtained a marked increase (up to 30 per cent) in total phenolic compounds (TPC) of the extracts after applying HPP. Seo et al.7 found that HPP assisted extraction (500 MPa / 60°C / 15 min) significantly increased the extraction yield of TPC from raspberry fruits compared to the conventional extraction, which involves the use of solvents and heating; especially higher concentrations of caffeic acid, catechin, and ferulic acid were measured in the HPP extract (in water) than in the water extract (extracted at 100ºC). Corrales et al.8 evaluated the effects of HPP on polyphenol extraction yield from grape by-products and found a significant increase (the percentage increase depended on the type of polyphenol) in the total and individual anthocyanin content after HPP in comparison to other extraction methods. Xi9 obtained a 17 per cent higher yield of lycopene from tomato paste waste with HPP-assisted (500 MPa / 25°C / 1 min) extraction in ethanol (75 per cent) compared to 30 minutes of ethanol extraction at 25°C and ambient pressure. The common conclusion in these studies and others is that HPP results in comparable or better recoveries and is more time and solvent efficient compared to traditional extraction methods. HPP is able to enhance mass transfer processes within plant cellular tissues, as the solubility of plant metabolites and permeability of cytoplasmatic membranes can be affected high pressure3.
The bioavailability and bioaccessibility of nutrients is highly dependent on the structure of the food matrix, and as HPP can enhance the extractability of, for example, carotenoids and phenolic compounds, it may favour the bioaccessibility and bioavailability of nutrients. However, there is no simple and direct correlation between process-induced matrix disruption and the extractability and bioavailability of nutrients. Indeed, studies, so far, indicate that the impact of processing, in general and HPP in particular, on the bioaccessibility and bioavailability of nutrients is dependent on the type of nutrient, the structure and composition of the food matrix and the processing technique employed.
Reduced allergenicity
Food allergies are increasing in developed countries and some evidence indicates that they are also increasing in developing countries, although these are less reported and investigated. The most frequently reported food allergies are to bovine milk, eggs, apples, peanuts, shellfish, soy and wheat. Most allergenic proteins, particularly plant allergens, appear to belong to a limited number of proteins with common characteristics that may render them allergenic. HPP can give rise to structural changes in proteins and, therefore, has been used to alter conformation and allergenic potential of food proteins. Furthermore, HPP has shown a potential to reduce the allergy risks of some foods without eliminating the allergenic proteins itself, but through extraction or release of membrane-bound allergens into the environment where they can be removed or attacked by hydrolytic enzymes. HPP has been reported to reduce the allergenic potential and risk of a number of products, primarily nuts, fruits and vegetables, dairy products and seafood.
Only a few studies have been conducted using HPP to modify or reduce the allergenic risk of peanuts and almonds, but found no change in allergenicity. Many studies reported reductions of extracted apple allergens after HPP based on immunoreactivity assays, although some of the reported reductions are not statistically significant. Scheibenzuber10 found important modifications in allergens and that 19 allergic patients tolerated HPP apple without symptoms of oral allergy syndrome. Moreover, prick to prick tests showed a correlation between apple allergen inactivation and applied pressure level. β-lactoglobulin (β-lg) is the major allergen in bovine milk and HPP (200-600 MPa / 20-68ºC / 10 min) of whey and skimmed milk significantly enhanced the antigenicity response of β-lg by 14-fold possibly due to weakening of non-covalent bonds under pressure resulting in a better accessibility for the antibodies11.
The increase in antigenicity may be associated with exposure of epitopes of the native protein molecule, which become accessible for antibodies after pressure-induced unfolding and aggregation. On the other hand, the HPP-induced conformational changes of the milk proteins can make enzymatic digestion easier, thereby enhancing the antigenicity. Chicón et al.12 successfully applied proteolytic enzymes (chymotrypsin and trypsin) during HP treatment (100 MPa / 20 min) of β-lg solutions and found a decrease of allergenic potential. Again, this reduction was explained by an increase of accessibility of potentially immunogenic hydrophobic regions to the enzyme, thereby resulting in an improved hydrolysis. Hence, HPP application during enzymatic hydrolysis of whey proteins can be used to rapidly prepare hypoallergenic foods possibly with increased sensory and nutritional attributes. Similarly, HPP (450 MPa / 40°C / 55 min) was used to diffuse one per cent saline containing papain protease into shrimp meat and the increased enzyme activity successfully hydrolysed allergens in the meat13. HPP treatment (300 MPa / 40°C / 15 min) resulted in a significant decrease in the allergenicity of soybean sprout proteins, while the nutritional value was largely retained14.
Preserving essential fatty acids and salt reduction
The promising application of HPP for the production of healthy food products is not limited to improved extraction of bioactive compounds or reducing allergenicity. The overall goal of HPP is to increase the range of food products that are safe and healthy. From a technological point of view this may mean to obtain minimally modified compounds or compounds with changed functionality. A change in functionality can have a positive health impact by preserving healthy lipids and avoiding unhealthy additives like salt and phosphates. Various studies on how to utilise HPP for manufacturing healthy food products have been reported.
Seafood contains healthy polyunsaturated lipids (PUFA) and is recommended in a healthy diet. However, seafood is also very sensitive towards various degradation reactions and processes resulting in a very short shelf-life of often less than seven days for fresh fish and shellfish. The hydrolysis of lipids by lipase enzymes results in free fatty acids (FFA), and of special concern are the PUFAs, which are highly reactive and easily oxidise, resulting in a lower nutritional value of the fish. Storage of pressurised (150, 300 MPa / 20°C / 15 min) Atlantic salmon at 4°C up to 6 days did not change the levels of n-3 and n-6 PUFAs or the saturated fatty acid contents compared to raw stored fish15. Cruz-Romero et al.16 found that HPP (at 260 (three min), 500 (five min), or 800 (five min) MPa/20°C) of oysters did not change the fatty acid profile compared to untreated oysters. They also reported that the n-3/n-6 ratios were numerically higher, though not statistically significant, in high pressure-treated oysters, which indicates increased availability of n-3 PUFAs, beneficial for human health. Milk is another important food product containing important nutraceuticals like essential FFAs, but unfortunately milk is also subjected to lipolysis resulting in nutritional degradation. A superior nutritional beverage combining milk and orange juice was subjected to HPP by Barba et al.17. It was found that PUFA content did not change due to pressure, while monosaturated FFA increased and saturated FFA increased at 100 and 200 MPa but decreased at 300 and 400 MPa. For a vegetable beverage (tomato, green pepper, green celery, cucumber, onion, carrot, lemon, and 0.8 per cent virgin olive oil), HPP had no effect on the three FFA groups17.
HPP has the potential to indirectly produce healthy foods by reducing the needs for unhealthy additives. Salts are the most criticised compounds in food and the concern of many European food regulatory agencies. In most food products sensory characteristics such as texture, juiciness, flavour, and taste as well as appearance, are the most important quality characteristics in relation to overall acceptability by consumers. The manufacturing of, for example, meat products often involve the use of salts and additives due to the beneficial textural and other sensory properties. Hence, the critical concern in reducing salt and other additives is the challenge to maintain sensory and functional attributes. Sikes et al.18 investigated the effect of HPP and simple salt reduction (NaCl concentrations of 0-2 per cent) on beef sausages. They found that HPP of the low-salt sausages reduced cooking losses and improved texture. Moreover, sensory panels reported a greater acceptability in both appearance and texture of the sausages. Grossi et al.19 found that with addition of carrot fibre or potato starch, it was possible to lower the salt content to 1.2 per cent (and no phosphates) in pressurised pork sausages. An important finding regarding the salt reduction was that the use of starch or fibre had more impact on textural properties than the level of salt and the water binding capacity was improved. These studies among others show that HPP reduces the need for fat, salt, and phosphate in meat products without altering and possibly improving quality.
Conclusion
It is clear from the foregoing discussion that HPP has a significant potential for improving the extraction yield of bioactive components from plant materials for use as ingredients in food as well as nutraceutical and pharmaceutical products. The potential application of HPP for reducing the allergenicity of certain food products and for the production of food products with reduced salt and fat content as well as improved fatty acid composition has also been demonstrated. It is believed that this knowledge will pave the way for the next generation of HPP food products and ingredients.
References
- Barba, F.J., Esteve, M.J., Frígola, A. (2012). High pressure treatment effect on physicochemical and nutritional properties of fluid foods during storage: A review. Comprehensive Reviews in Food Science and Food Safety, 11(3), 307 – 322.
- Sanchez-Moreno, C., De Ancos, B., Plaza, L., Elez-Martinez, P., Cano, M.P. (2009). Nutritional approaches and health-related properties of plant foods processed by high pressure and pulsed electric fields. Critical Reviews in Food Science and Nutrition, 49(6), 552 – 576.
- Xi, J. (2013). High-Pressure Processing as Emergent Technology for the Extraction of Bioactive Ingredients From Plant Materials. Crit. Rev. Food Sci. Nutr. 53, 837–852.
- Huang, H-W., Hsu, C-P., Yang, BB., Wang, C-Y. (2014). Potential utility of high-pressure processing to address the risk of food allergen concerns. Comprehensive Reviews in Food Science and Food Safety, 13, 78-90.
- Gustavsson, J., Cederberg, C., Sonesson, U., van Otterdijk, R., & Meybeck, A. (2011). Global food losses and food waste. Extend, causes and prevention. Rome: Food and Agriculture Organization of the United Nations. http://www.fao.org/fileadmin/user_upload/ags/publications/GFL_web.pdf
- Jun X, Deji S, Zhao S, Lu B, Li Y, Zhang R. (2009). Characterization of polyphenols from green tea leaves using a high hydrostatic pressure extraction. Int. J. Pharm. 382 (1–2): 139-143.
- Seo YC, Choi WY, Kim JS, Yoon CS, Lim HW, Cho JS, Ahn JH, Lee HY. (2011). Effect of ultra high pressure processing on immuno-modulatory activities of the fruits of Rubus coreanus Innov Food Sci Emerg Technol 12: 207–215.
- Corrales M, García AF, Butz P, Tauscher B. (2009). Extraction of anthocyanins from grape skins assisted by high hydrostatic pressure. J Food Eng 90(4): 415-421.
- Xi, J. (2006). Application of high hydrostatic pressure processing of food to extracting lycopene from tomato paste waste. High Pressure Res. 26(1):33–41.
- Scheibenzuber M. (2003). Molekulare and klinische Auswirkungen einer Hochdruckbehandlung allergener Lebensmittel. PhD thesis, TU Muenchen.
- Kleber N, Maier S, Hinrichs J. (2007). Antigenic response of bovine β-lactoglobulin influenced by ultra-high pressure treatment and temperature. Innov Food Sci Emerg Technol. 8: 39–45.
- Chicón R, López-Fandiño R, Alonso E, Belloque J. (2008). Proteolytic pattern, antigenicity, and serum immunoglobulin E binding of beta-lactoglobulin hydrolysates obtained by pepsin and high-pressure treatments. J Dairy Sci. 91(3): 928-38.
- Xie & Hu (2013). Patent; Preparing shrimp meat, by removing shrimp head, tail and intestinal line, removing membrane on surface of meat, grinding and introducing meat in salt water, adding papain powder, and maintaining mixture at certain temperature.
- Penas E, Gomez R, Frias J, Baeza ML, Vidal-Valverde C. (2011). High hydrostatic pressure effects on immunoreactivity and nutritional quality of soybean products. Food Chem., 125:423–9.
- Yagiz Y, Kristinsson HG, Balaban MO, Welt BA, Ralata M, Marshall MR. (2009). Effect of high pressure processing and cooking treatment on the quality of Atlantic salmon. Food Chem 116: 828-8356.
- Cruz-Romero MC, Kerry JP, Kelly AL. (2008). Fatty acids, volatile compounds and colour changes in high-pressure-treated oysters (Crassostrea gigas). Innov Food Sci Emerg Technol 9: 54-61.
- Barba FJ, Esteve MJ, Frigola A. (2012). Impact of high-pressure processing on vitamin E (α-, γ-, and d-tocopherol), vitamin D (cholecalciferol and ergocalciferol), and fatty acid profiles in liquid foods. J Agric Food Chem 60: 3763-3768.
- Sikes AL, Tobin AB, Tume RK. (2009). Use of high pressure to reduce cook loss and improve texture of low-salt beef sausage batters. Innov Food Sci Emerg Technol 10:405-412.
- Grossi A, Søltoft-Jensen J, Knudsen JC, Christensen M, Orlien V. (2012). Reduction of salt in pork sausages by the addition of carrot fibre or potato starch and high pressure treatment. Meat Sci 92(4): 481-489.
About the authors
Vibeke Orlien has an MSc in mathematics and chemistry and a PhD degree in food chemistry. Since 2008, Vibeke has been employed as an Associate Professor in Food Chemistry at the Department of Food Science, Faculty of Life Sciences, University of Copenhagen. From 2010 Vibeke has been Head of the Food Chemistry Research Section at the Department of Food Science. Her major research area is high pressure processing of food and the effects on food components and their properties.
Francisco J. Barba holds a European PhD in Food Science and Technology from the University of Valencia and also holds degrees in Pharmacy and in Food and Technology. He is Assistant Professor in Food Science and Nutrition Area, Faculty of Pharmacy, University of Valencia. His research focus is on non-conventional processing for preservation and/or extraction of bioactive compounds from food. His major research areas are the recovery of bioactive compounds by using non-conventional methods and evaluating the effects of emerging technologies on nutritional and quality parameters of liquid foods.
Roman Buckow holds an MSc and PhD in Food Process Engineering from the Berlin Institute of Technology, Germany. In 2006, Roman joined Food Science Australia (now CSIRO) to complete his postdoctoral research fellowship. He soon became a Research Group Leader and is currently a Stream Leader focusing on the development of technology platforms for improving agri-food product quality and value. Roman’s research interests include sustainable and healthy food manufacture through conventional and novel food processing technologies, including high pressure processing.
Netsanet Shiferaw Terefe has a BSc in chemical engineering, a Master’s degree in food engineering and a PhD in bioscience engineering from the Catholic University of Leuven, Belgium. She is currently a Research Scientist at CSIRO Animal, Food and Health Sciences (CAFHs) working on emerging food processing technologies such as high pressure, pulsed electric field and ultrasonic processing and extraction and separation technologies for value addition to food processing waste.
I find your article very interesting. I have a packaging background and 12 years experience in HPP. Your article very factual. One of the first lessons I learned a long time ago was after the first test the customer has to go back and reformulate.
Besides the reduction in seasonings HPP intensifies or greatly enhances the flavor. Minimally an all natural or organic product will have the product life cycle extended by a factor of 3.
The percentage of manufacturers that understand the full effect of HPP is very small. If you have any questions or would like to discuss this further please feel free to contact me. Thank you.
Regards,
GaryD
hi Gary DesLauriers..
M looking for the recent research being done in the field of high pressure technology.
Can u please tell me the developments in the process design in this field.
my email in [email protected].
looking forward for your reply.